Anticancer mechanisms of cannabinoids
Curr Oncol. 2016 Mar; 23(Suppl 2): S23–S32.
Published online 2016 Mar 16. doi: 10.3747/co.23.3080
G. Velasco, PhD,*†‡ C. Sánchez, PhD,*§ and M. Guzmán, PhD*†‖
Abstract
In addition to the well-known palliative effects of cannabinoids on some cancer-associated symptoms, a large body of evidence shows that these molecules can decrease tumour growth in animal models of cancer. They do so by modulating key cell signalling pathways involved in the control of cancer cell proliferation and survival. In addition, cannabinoids inhibit angiogenesis and decrease metastasis in various tumour types in laboratory animals. In this review, we discuss the current understanding of cannabinoids as antitumour agents, focusing on recent discoveries about their molecular mechanisms of action, including resistance mechanisms and opportunities for their use in combination therapy. Those observations have already contributed to the foundation for the development of the first clinical studies that will analyze the safety and potential clinical benefit of cannabinoids as anticancer agents.
Keywords: Cannabinoids, apoptosis, autophagy, cell proliferation, angiogenesis, cell signalling, combination therapy
INTRODUCTION
Preparations of Cannabis sativa L. (marijuana) have been used for many centuries both medicinally and recreationally. However, the chemical structures of their unique active components—the cannabinoids—were not elucidated until the 1960s. Three decades later, the first solid clues to cannabinoid molecular action were established, which led to an impressive expansion of basic cannabinoid research and a renaissance in the study of the therapeutic effects of cannabinoids in various fields, including oncology. Today, it is widely accepted that, of the approximately 108 cannabinoids produced by C. sativa, Δ9-tetrahydrocannabinol (thc) is the most relevant because of its high potency and abundance in plant preparations1,2.
Tetrahydrocannabinol exerts a wide variety of biologic effects by mimicking endogenous substances—the endocannabinoids anandamide3 and 2-arachidonoylglycerol4,5—that engage specific cell-surface cannabinoid receptors6. So far, two major cannabinoid-specific receptors—cb1 and cb2—have been cloned from mammalian tissues and characterized7,8. In addition, other receptors such as the transient receptor potential cation channel subfamily V, member 1, and the orphan G protein–coupled receptor 55 have been proposed to act as endocannabinoid receptors6. Most of the effects produced by cannabinoids in the nervous system and in non-neural tissues rely on cb1 receptor activation. In contrast, the cb2 receptor was initially described to be present in the immune system6, but was more recently shown to also be expressed in cells from other origins9,10. Notably, expression of the cb1 and cb2 receptors has been found in many types of cancer cells, but not necessarily correlating with the expression of those receptors in the tissue of origin9,11,12.
The endocannabinoids, together with their receptors and the proteins responsible for their synthesis, transport, and degradation, constitute the endocannabinoid system. Aside from its pivotal neuromodulatory activity13, the endocannabinoid system exerts other regulatory functions in the body such as control of cardiovascular tone, energy metabolism, immunity, and reproduction14,15. This miscellaneous activity makes the pharmacologic manipulation of the endocannabinoid system a promising strategy for the management of many diseases. Specifically, cannabinoids are well known to exert palliative effects in cancer patients14,15. Their best-established use is the inhibition of chemotherapy-induced nausea and vomiting15,16. Today, capsules of thc (Marinol: AbbVie, North Chicago, IL, U.S.A.) and its synthetic analogue nabilone (Cesamet: Meda Pharmaceuticals, Somerset, NJ, U.S.A.) are approved for that purpose. Cannabinoids also inhibit pain, and thus a standardized cannabis extract (Sativex: GW Pharmaceuticals, Salisbury, U.K.) has already been approved in Canada and is currently the subject of large-scale phase iii clinical trials for managing cancer-associated pain. Another potential palliative effect of cannabinoids in oncology, supported by phase iii clinical trials, includes appetite stimulation and attenuation of wasting. In that respect, Marinol can currently be prescribed for anorexia associated with weight loss in aids patients.
The therapeutic potential of cannabinoids in oncology might not be restricted to their aforementioned palliative actions. Thus, numerous studies have provided evidence that thc and other cannabinoids exhibit antitumour effects in a wide array of animal models of cancer12,16,17. Moreover, those observations led to the development of several clinical studies to investigate the antitumour activity of thc in humans (see “Clinical Antitumour Effects of Cannabinoids” later in this article). Nonetheless, a few studies have shown that, under certain conditions, cannabinoid treatment can stimulate cancer cell proliferation in vitro18,19 and interfere with the tumour-suppressor role of the immune system20,21. Likewise, reports about the role of the endocannabinoid system in cancer (tumour-suppressor or oncogenic) are conflicting22.
Reports concerning the biologic role of the endocannabinoid system in cancer physiopathology are sparse. Although some exceptions that might be tumour-type-specific are known, cannabinoid receptors and their endogenous ligands are both generally upregulated in tumour tissue compared with non-tumour tissue16,22–24. Additionally, various studies have associated the expression levels of cannabinoid receptors, endocannabinoids, or endocannabinoid-metabolizing enzymes with tumour aggressiveness22,25,26, which suggests that the endocannabinoid system might be overactivated in cancer and hence pro-tumourigenic22. In support of that hypothesis, reports showed that genetic ablation of cb1 and cb2 receptors in mouse models of cancer reduces ultraviolet light–induced skin carcinogenesis27, that overexpression of cb2 receptor enhances the predisposition to leukemia after leukemia viral infection28, and that cb2 promotes her2 (human epidermal growth factor receptor 2) pro-oncogenic signalling in breast cancer29.
Conversely, and in line with the evidence supporting the hypothesis that pharmacologic activation of cannabinoid receptors decreases tumour growth12,16, upregulation of endocannabinoid-degrading enzymes has been observed in aggressive human tumours and cancer cell lines25,26, indicating that endocannabinoid signalling can also have a tumour-suppressive role. In support of that observation, deletion of cb1 receptors was noted to accelerate intestinal tumour growth in a genetic mouse model of colon cancer30, increased endocannabinoid levels were observed to diminish azoxymethane-induced precancerous lesions in the mouse colon31, and reduction in the expression of the endocannabinoid-degrading enzyme monoacylglycerol lipase was seen to decrease tumour growth in xenografted mice25.
Further studies—including analyses of the activation of the precise signalling mechanisms involved in the regulation of cannabinoid-induced cell death, and of cell proliferation upon genetic or pharmacologic manipulation of the endocannabinoid system—are therefore needed to clarify the contextual determinants that result in the system acting as either a guardian or an inducer of tumourigenesis or tumour progression. The present review summarizes such observations and provides an integrated view of the molecular mechanisms responsible for cannabinoid antitumour activity. It also discusses the experimental evidence supporting the existence of mechanisms of resistance to the cell death–promoting actions of thc in certain types of cancer cells, the strategies that could possibly be used to overcome such resistance, and the preclinical data supporting the potential usefulness of the combined administration of cannabinoids and other drugs in anticancer therapies.
PRECLINICAL ANTITUMOUR ACTIVITY
Since the late 1990s, a large body of evidence has accumulated demonstrating that various cannabinoids exert antitumour effects in a wide variety of experimental models of cancer, ranging from cancer cell lines in culture to genetically-engineered mice (reviewed by Velasco et al.17). Multiple cannabinoids have shown this activity, including thc; the endocannabinoids 2-arachidonoylglycerol and anandamide; and various synthetic cannabinoid receptor agonists that have either comparable affinity for the cb1 and cb2 receptors (for example, WIN 55,212-2 or HU-210), a higher affinity for cb1 (for example, methanandamide), or a higher affinity for cb2 (for example, JWH-133). Those findings strongly support that, aside from the role played by the endogenous cannabinoid system in cancer, pharmacologic stimulation of cb receptors is, in most cases, antitumourigenic. Nonetheless, a few reports have proposed a tumour-promoting effect of cannabinoids18–21. Those apparently conflicting observations are discussed in the next subsection.
Mechanisms of Antitumour Effects
Cannabinoids impair tumour progression at various levels. Their most prevalent effect is the induction of cancer cell death by apoptosis and the inhibition of cancer cell proliferation. At least one of those actions has been demonstrated in almost all cancer cell types tested17. In addition, in vivo experiments have shown that cannabinoids impair tumour angiogenesis and block invasion and metastasis.
Induction of Cancer Cell Death
A significant amount of the research conducted so far on the mechanism of cannabinoid antitumour activity has focussed on glioma cells. Initial studies showed that thc and other cannabinoids induce the apoptotic death of glioma cells by cb1- and cb2-dependent stimulation of the de novo synthesis of the pro-apoptotic sphingolipid ceramide23,32–34. Further studies based on the analysis of the gene expression profile of thc-sensitive and -resistant glioma cells yielded further insight into the specific signalling events downstream of ceramide that are activated in cancer cells by cannabinoids35. Thus, it was found that treatment with thc results in enhanced expression of the stress-regulated protein p8 (nupr1), a transcriptional regulator that has been implicated in the control of tumourigenesis and tumour progression36, together with several of its downstream targets, such as the endoplasmic reticulum (er) stress–related transcription factors atf4 and chop, and the pseudokinase tribbles homologue 3 (trib3)35. This thc-triggered stimulation of the p8-regulated pathway (Figure 1) enhances the inhibitory interaction of trib3 with a pro-survival kinase, akt37,38, which leads to inhibition of the mammalian target of rapamycin complex 1 (mtorc1) and the subsequent stimulation of autophagy-mediated cell death37.
Cannabinoid-induced apoptosis relies on the stimulation of endoplasmic reticular (ER) stress and autophagy. Here, the mechanism of cannabinoid-induced apoptosis in glioma, pancreatic, and hepatocellular carcinoma cells is depicted. This signalling route could constitute the main mechanism of cannabinoid-induced cell death, with some variations inherent to different types of cancer cells. CB1 = cannabinoid CB1 receptor; CB2 = cannabinoid CB2 receptor; SPT = serine palmitoyltransferase; elF2α = eukaryotic translation initiation factor 2α; P inscribed in a circle = protein phosphorylation upon treatment with Δ9-tetrahydrocannabinol (THC); ATF-4 = activating transcription factor 4; CHOP = C/EBP homologous protein; AKT = protein kinase B; TRIB3 = tribbles-homologue 3; mTORC2 = mammalian target of rapamycin complex 2; CDKs = cyclin-dependent kinases; pRb = retinoblastoma protein; CaCMKKβ = calcium/calmodulin–dependent protein kinase kinase 2β; AMPK = AMP-activated protein kinase.
Autophagy is an essential cellular process participating in a number of physiologic functions within the cell39,40. During autophagy, organelles and other cytoplasmic components are engulfed within double-membrane vesicles called “autophagosomes.” The maturation of those vesicles involves their fusion with lysosomes, which leads in turn to the degradation of the autophagosome components by lysosomal enzymes40. Autophagy is primarily a cytoprotective mechanism, although its activation can also lead to cell death40–42.
Cannabinoids induce autophagy in various types of cancer cells in culture, and pharmacologic or genetic inhibition of autophagy prevents cannabinoid antitumour action in various animal models of cancer (Figure 1), thus demonstrating that autophagy is important for cannabinoid antineoplastic activity37,43,44. Moreover, autophagy blockade prevents cannabinoid-induced apoptosis and cell death, whereas apoptosis blockade prevents cannabinoid-induced cell death, but not autophagy37,43,44. Those observations indicate that autophagy is upstream of apoptosis in the mechanism of cannabinoid-induced cell death (Figure 1).
The importance of this pathway is highlighted by the ability of various chemical and genetic manipulations to block cannabinoid-induced cell death. In hepatocellular carcinoma cells, the cannabinoid-evoked and erstress–dependent activation of calcium/calmodulin-dependent protein kinase kinase 2β and amp-activated protein kinase leads, together with the p8–trib3 pathway, to autophagy and apoptosis43. The cannabinoid-evoked inhibition of akt was able to promote cycle arrest in breast cancer and melanoma cells, as well as apoptosis through additional mechanisms, including decreased phosphorylation of the pro-apoptotic protein bcl2-associated agonist of cell death45 and activation of the cyclin-dependent kinase inhibitory proteins p21 and p2724,46,47, leading to the subsequent decreased phosphorylation of the retinoblastoma protein, which would thus arrest the cell cycle.
The direct participation of the p8/trib3–mediated autophagy pathway in the antitumour action of cannabinoids has been clearly demonstrated in glioma cells, pancreatic and hepatic cancer cells, and melanoma cells35,37,43,44,48,49. At least part of that signalling route has also been found to be upregulated with cannabinoid treatment in other types of cancer cells, an observation which suggests that—with some variations—this pathway could be a general mechanism by which activation of cb1 and cb2 receptors promotes cancer cell death.
Additional mechanisms might nonetheless cooperate with the p8/trib3–mediated autophagy pathway to evoke cancer cell death (Figure 1). For example, in hepatocellular carcinoma cells, thc and the cb2 receptor agonist JWH-015 can trigger an er stress–dependent activation of amp-activated protein kinase that cooperates with the trib3-mediated inhibition of the akt–mtorc1 axis in the stimulation of autophagy-mediated cell death44. In melanoma46, breast carcinoma24,50, and prostate carcinoma51 cells, cannabinoids can induce cell-cycle arrest in concert with apoptosis24,46,51. Notably, cannabinoid antiproliferative action—at least in melanoma46 and breast cancer24 cells—also relies on akt inhibition.
Likewise, the effect of cannabinoids in hormone- dependent tumours might rely, at least in part, on the ability to interfere with the activation of growth factor receptors12,16. Some of those and other mechanisms52 might participate, together with the autophagy-mediated cell death pathway, in the cytotoxic action of cannabinoids in various types of cancer cells. However, further investigation is required to clarify the issue.
Research conducted during the last few years has shed light on the intracellular signalling mechanisms underlying cannabinoid anticancer action. However, a number of important observations—in particular, those related to the role played by cannabinoid receptors in the triggering of the signals—remain to be clarified. For example, in contrast to the death-promoting action of cannabinoids on cancer cells, the viability of normal (non-transformed) cells is unaffected or, under certain conditions, even enhanced by cannabinoid challenge33–35,37,53. For example, thc treatment of astrocytes (a cell type that expresses functional cb1 receptors) does not trigger the activation of er stress, upregulation of the p8 pathway, inhibition of the akt–mtorc1 axis, or stimulation of autophagy and apoptosis, even when concentrations of thc higher than those that promote glioma cell death are used35,37. Similar results were obtained for primary embryonic fibroblasts35,41 and other types of non-transformed cells expressing functional cannabinoid receptors in comparison with their transformed counterparts24,46,54,55. Thus, stimulation of cannabinoid receptors seems to be coupled with the activation of different signalling mechanisms in transformed and non-transformed cells. The precise molecular reasons for this variation in behaviour remain as an important open question in the cannabinoid field. Another intriguing observation is that, in some types of cancer cells (such as glioma cells), pharmacologic blockade of either cb1 or cb2 prevents cannabinoid-induced cell death with similar efficacy33,56, and yet in other types of cancer cells (pancreatic48, breast24, or hepatic43 carcinoma cells, for example), antagonists of cb2 but not of cb1 inhibit cannabinoid antitumour actions. The reason that cannabinoids produce their antitumour actions through one or the other of these receptor types depending on the type of cancer cell has yet to be established.
Some cannabinoid receptor agonists promote cancer cell death more efficiently than other agonists that exhibit similar or even higher affinity for the cb1 or cb2 receptors. For example, thc promotes cancer cell death in a cb1- or cb2-dependent manner (or both) at lower concentrations than does the synthetic cannabinoid receptor agonist WIN 55,212-2, although the latter agent shows significantly higher affinity for cb1 and cb2 in binding assays6.
Further work aimed at investigating, for example, cb receptor homo- or hetero-oligomerization in response to various cannabinoid agonists, their associations with specific domains in the plasma membrane such as lipid rafts, changes in the subcellular location of cb receptors, and the selective coupling to various G proteins and other signalling proteins, will be essential to answer the foregoing questions and to precisely define the role played by each cannabinoid receptor type as an anticancer signalling platform.
Notably, cannabidiol (cbd), a phytocannabinoid with a low affinity for cannabinoid receptors15, and other marijuana-derived cannabinoids57 have also been proposed to promote the apoptotic death of cancer cells acting independently of the cb1 and cb2 receptors. The mechanism by which cbd produces this effect has not as yet been completely clarified, but it seems to rely—at least in part—on its ability to enhance the production of reactive oxygen species in cancer cells58,59. It has also been proposed that cbd might activate trpv2 receptors to promote glioma cell death60.
Inhibition of Angiogenesis, Invasion, and Metastasis
In cancer cells, cannabinoids block the activation of the vascular endothelial growth factor (vegf) pathway, an inducer of angiogenesis. Specifically, various elements of the cascade, such as the main ligand (vegf) and the active forms of its main receptors (vegfr1 and vegfr2), are downregulated with cannabinoid treatment of skin carcinomas54, gliomas32,61, and thyroid carcinomas62. In vascular endothelial cells, cannabinoid receptor activation inhibits proliferation and migration, and induces apoptosis61,63. Those and perhaps other cannabinoid-evoked actions result in a normalized tumour vasculature—that is, smaller and fewer vessels that are more differentiated and less leaky.
Likewise, cb1 or cb2 receptor agonists (or both) reduce the formation of distant tumour masses in animal models of both induced and spontaneous metastasis, and inhibit adhesion, migration, and invasiveness of glioma64, breast65,66, lung67,68, and cervical68 cancer cells in culture. Those effects depend, at least in part, on the modulation of extracellular proteases (such as matrix metalloproteinase 2)64 and their inhibitors (such as tissue inhibitor of matrix metalloproteinases 1)68.
Notably, pharmacologic inhibition of ceramide biosynthesis abrogates the antitumour and antiangiogenic effect of cb1 or cb2 receptor agonists (or both) in glioma xenografts, and decreases vegf production by glioma cells in vitro and in vivo32. Likewise, inhibition of matrix metalloproteinase 2 expression and glioma cell invasion is prevented by blocking ceramide biosynthesis and by knocking down p8 expression64. Although further research is still necessary to precisely define the molecular mechanisms responsible for those actions of cannabinoids, the observations indicate that the ceramide/p8–regulated pathway plays a general role in the antitumour activity of cannabinoids targeting cb1 and cb2.
It is worth noting that cbd, by acting independently of the cb1 and cb2 receptors, produces a remarkable anti-tumour effect—including reduction of invasiveness and metastasis—in various animal models of cancer. This effect of cbd seems to rely, at least in part, on the downregulation of the helix-loop-helix transcription factor inhibitor of dna binding 169,70.
Regulation of Antitumour Immunity
Notably, stimulation of cannabinoid receptors can lead to important changes in the processes that regulate anti-tumour immunity. Thus, for example, treatment of mice with thc triggers a shift (from Th1 to Th2) of cytokine profile20,71–73 and induces mobilization of myeloid-derived suppressor cells74, two events that play a critical role in the suppression of antitumour immunity. In agreement with that notion, stimulation of cb2 has been proposed in some reports to enhance tumourigenesis by interfering with tumour surveillance by the immune system20,21.
By contrast, cannabinoids can also enhance immune system–mediated tumour surveillance in some contexts: the antitumour action of WIN 55,212-2 (a mixed cb1 or cb2 agonist) or JWH-133 (a cb2-selective agonist) was more pronounced in melanoma xenografts generated in immunocompetent mice than in those generated in immunodeficient mice46, a finding which also indicates that, at least in this model, stimulation of cb2 inhibits tumour growth primarily through direct effects on cancer cells rather than necessarily by interfering with the normal antitumour function of the immune system. In line with that idea, treatment of immunocompetent rats with very high doses of thc (50 mg/kg daily 5 times per week) for 2 years lowered the incidence of several types of tumours and enhanced the overall survival of the animals55. Those observations might be related to the ability of thc to reduce inflammation75,76, an effect that might prevent certain types of cancer76,77.
For cannabinoid use to be clinically successful, anti-tumour effects will have to overcome immunosuppressive (potentially tumour-promoting) effects. Additional studies should clarify the issue. For example, it could be conceivable to study the effect of cannabinoid administration on the generation and progression of tumours with varying sensitivity to cannabinoids and generated in immunocompetent or immunodeficient mice in which the expression of cb1 or cb2 receptors (or both) in cells from the immune system has been genetically manipulated.
RESISTANCE MECHANISMS
Numerous studies have contributed to an appreciation of the heterogeneity of cancer, whereby each subtype of cancer—and even each individual tumour—exhibits a series of molecular characteristics that determines its behaviour and, in particular, its responsiveness to various anticancer drugs. In agreement with that line of reasoning, a recent report investigated the molecular features associated with the resistance of a collection of human glioma cell lines and primary cultures to cannabinoid antitumour action56. The study showed that, although the apoptotic effect of thc on glioma cells relied on the stimulation of cannabinoid receptors and activation of the p8-mediated autophagy pathway, the differences in the sensitivity to thc-induced cell death correlated with enhanced expression of a particular set of genes in the thc-resistant glioma cells rather than with the presence of different expression levels of cb1 or cb2 receptors56. Interestingly, upregulation of one of those genes, midkine (MDK), which encodes a growth factor that was previously associated with increased malignancy and resistance to anticancer therapies in several types of tumours77,78, correlates with lower overall survival in patients with glioblastoma56. Moreover, mdk plays a direct role in the resistance to thc action through stimulation of anaplastic lymphoma kinase (alk79). Thus, the stimulation of alk by mdk inhibits the thc-evoked autophagy-mediated cell-death pathway. Further research should clarify whether that mechanism could also be responsible for the resistance to other therapies of cancer cells expressing high levels of mdk. Interestingly, in vivo silencing of MDK or pharmacologic inhibition of alk in a mouse xenograft model abolishes the resistance to thc treatment of established tumours derived from cannabinoid-resistant glioma cells56.
Taken together, the foregoing findings support the idea that stimulation of the mdk–alk axis promotes resistance to thc antitumour action in gliomas and could help to set a foundation for the potential clinical use of thc in combination with inhibitors of the mdk–alk axis (Figure 2). Glioblastoma is highly resistant to current anticancer therapies80–82. Specifically, resistance of glioma cells to cannabinoid-induced cell death relies, at least in part, on enhanced expression of mdk and the subsequent activation of alk56. Likewise, enhanced expression of the heparin-bound epidermal growth factor receptor (egfr) ligand amphiregulin can promote resistance to thc antitumour action by stimulation of extracellular signal-regulated kinase (erk)83. The combination of thc with pharmacologic inhibitors of alk (or genetic inhibition of MDK) enhances cannabinoid action in resistant tumours, which provides a rationale for the design of targeted therapies capable of increasing cannabinoid antineoplastic activity56. Combinations of cannabinoids with classical chemotherapeutic drugs such as the alkylating agent temozolomide (the benchmark agent for the management of glioblastoma80,84) have been shown to produce a strong anticancer action in animal models85. Combining cannabinoids and temozolomide is thus a very attractive possibility for clinical studies aimed at investigating cannabinoid antitumour effects in glioblastoma. Other potentially interesting strategies to enhance cannabinoid anticancer action (still requiring additional experimental support from data obtained using preclinical models) could be to combine cannabinoids with er stress or autophagy inducers (or both) or with inhibitors of the mtorc1 axis.
Possible strategies for optimizing cannabinoid-based therapies against gliomas. GF = growth factors; Abs = antibodies; MDK = growth factor midkine; VEGF = vascular endothelial growth factor; RTK = receptor tyrosine kinase; ALK = anaplastic lymphoma receptor tyrosine kinase; EGFR = epidermal growth factor receptor; CB1 = cannabinoid CB1 receptor; CB2 = cannabinoid CB2 receptor; AREG = amphiregulin; ER stress = endoplasmic reticular stress; ERK = extracellular signal-regulated kinase; TMZ = temozolomide; TRIB3 = tribbles-homologue 3; AKT = protein kinase B; mTORC1 = mammalian target of rapamycin complex 1.
In line with that idea, alk inhibitors have started to be used in clinical trials for the management of non-small-cell lung cancer and other types of tumours86,87. Future research should clarify whether this mechanism of resistance to cannabinoid action operates in other types of tumours. In agreement with that possibility, MDK silencing enhanced the sensitivity of cannabinoid-resistant pancreatic cancer cells to thc-induced cell death56.
The release by cancer cells of other growth factors has also been implicated in the mechanism of resistance to cannabinoid antitumour action. Thus, increased expression of amphiregulin is associated with enhanced resistance to thc antitumour action in glioma xenografts83. Notably illustrating how the dose of cannabinoids could be crucial for optimal therapeutic effect, low (submicromolar) concentrations of thc or other synthetic cannabinoid agonists enhance the proliferation of several cancer cell lines in vitro. That effect relies on activation of the protease adam17, the shedding of heparin-bound egfr ligands including amphiregulin and the subsequent stimulation of the erk and akt pathways19. In line with that idea, a recent report showed that treatment with the synthetic cannabinoid CP 55,940 increases the proliferation of murine glioma cells engineered to express cb1 or cb2 receptors only when those receptors are coupled to akt activation18. Although a pro-tumourigenic effect has not been observed for the growth of tumour xenografts generated with glioma cells and treated with low doses of thc85, increased expression of amphiregulin promotes resistance to thc antitumour action through a mechanism that involves the egfr-dependent stimulation of erk and the subsequent inhibition of p8 and trb3 expression. Likewise, pharmacologic inhibition of egfr, erk83, or akt enhances the cell-death-promoting action of thc in glioma cultures (unpublished observations by the authors), which suggests that targeting egfr and the akt and erkpathways could enhance the antitumour effect of cannabinoids.
CANNABINOID-BASED COMBINATION THERAPIES
The use of combinational anticancer therapies has a number of theoretical advantages over single-agent strategies, because they allow for the simultaneous targeting of tumour growth, progression, and spread at various levels. In line with that idea, recent observations suggest that the combined administration of cannabinoids with other anticancer drugs acts synergistically to reduce tumour growth. For example, the administration of thc and temozolomide exerts strong antitumour action in glioma xenografts, an effect that is also evident in temozolomide-resistant tumours85. A similar effect was observed when thc and cbdwere combined with radiotherapy in animal models of glioma. Interestingly, no toxicity was observed in mice treated with combinations of thc and temozolomide85. Because most patients with glioblastoma undergo temozolomide treatment, the foregoing findings indicate that the combined administration of temozolomide and cannabinoids could be therapeutically exploited for the management of glioblastoma (Figure 2) and perhaps other tumour types such as melanoma44.
Likewise, another study recently showed that the combined administration of gemcitabine (the benchmark agent for the treatment of pancreatic cancer) and various cannabinoid agonists synergistically reduced the viability of pancreatic cancer cells88. Other reports indicated that anandamide and HU-210 might also enhance the anticancer activity of paclitaxel89 and 5-fluorouracil90 respectively.
An additional approach has been to combine thc with cbd, a phytocannabinoid that reduces (although to a lower extent than thc) the growth of several types of tumour xenografts through a still poorly-defined mechanism59,91,92. Combined administration of thc and cbd enhances the anticancer activity of thc and reduces the dose of thc needed to induce its tumour growth-inhibiting activity85,93. Moreover, the combination of thc and cbd together with temozolomide produces a striking reduction in the growth of glioma xenografts even when low doses of thc are used85. Likewise, the combination of thc, cbd, and radiotherapy also produced clear anticancer activity in an orthotopic model of glioma94. Notably, cbd was also shown to alleviate some of the undesired effects of thc administration such as convulsions, discoordination, and psychotic events, thus improving the tolerability of cannabis-based medicines15. As mentioned earlier, C. sativa produces approximately 108 different cannabinoids, and apart from cbd, some of the other cannabinoids present in marijuana might attenuate the psychoactive side effects of thc or even produce other therapeutic benefits15. Thus, we think that clinical studies aimed at analyzing the efficacy of cannabinoids as antitumour agents should be based on the use both of pure substances, such as thc and cbd, and of cannabis extracts containing controlled amounts of thc, cbd, and other cannabinoids.
CLINICAL ANTITUMOUR EFFECTS OF CANNABINOIDS
The clinical approval of cannabinoids is largely restricted to palliative uses in various diseases, but since the emergence of promising preclinical data, the antitumour effects of cannabinoids are beginning to be clinically assessed.
In a pilot phase i clinical study, 9 patients with actively- growing recurrent glioblastoma for whom standard therapy had previously failed underwent intracranial thc administration11. Under those conditions, cannabinoid delivery was safe and could be achieved without significant unwanted effects. In addition, although no statistically significant conclusions can be extracted from a cohort of 9 patients, the results obtained in the study suggest that some patients responded—at least partially—to thc treatment in terms of a decreased tumour growth rate as evaluated by magnetic resonance imaging11. Importantly, analyses of samples obtained from 2 study patients before and after thc administration indicated that the molecular mechanism of cannabinoid antitumour action—namely, p8 and trib3 upregulation35,37, mtorc1 inhibition37, stimulation of autophagy and apoptosis11,35,37, inhibition of cell proliferation11, decreased vegf signalling32, and matrix metalloproteinase 2 downregulation64 (delineated here earlier)—also operates in vivo.
Those findings were encouraging and reinforced interest in the potential use of cannabinoids in cancer therapies. However, they also highlighted the need for further research aimed at optimizing the use of cannabinoids in terms of patient selection, combination with other anti-cancer agents, and use of other routes of administration.
Administration of endocannabinoids or inhibitors of endocannabinoid-degrading enzymes has been shown to reduce the growth of various tumour xenograft types95,96 and could therefore be a reasonable strategy for targeting cannabinoid receptors for anticancer purposes. However, as discussed here earlier, the role of the endocannabinoid system, including the endocannabinoid-degrading enzymes, in the control of tumour generation and progression is not well understood. Because enhancing endocannabinoid tone only has mild antitumour effects in mice and because no inhibitor of endocannabinoid degradation has yet been approved for use in humans, clinical studies aimed at analyzing the efficacy of cannabinoids as antitumour agents should be based on the use of plant-derived or synthetic agonists of cannabinoid receptors rather than on endocannabinoids or inhibitors of endocannabinoid degradation.
The long-known therapeutic properties of C. sativa—including amelioration of symptoms associated with cancer and its chemotherapy—have led to the authorization of the medical use of the plant and its extracts in several countries. As already mentioned, C. sativa produces approximately 108 different cannabinoids, including thc and cbd. Some of the other cannabinoids present in marijuana might contribute to the attenuation of thc’s psychoactive side effects or even to the production of other therapeutic benefits15. However, pure drugs are more prone to standardization than complex molecular cocktails. Thus, it would be ideal for studies aiming to investigate the anticancer actions of cannabinoids in patients to be performed comparatively with both pure substances and cannabis extracts containing controlled amounts of thc, cbd, and other cannabinoids.
The most widely used route of administration for recreational and self-medicating marijuana is smoking. Although thc and other phytocannabinoids are rapidly absorbed by inhalation, smoking is an unattractive clinical option. Preclinical work in animal models has typically used peri-tumoural administration of cannabinoids. Likewise, in the only clinical trial in which a cannabinoid was assayed as an antitumour agent, thc was administered locally (intracranial delivery to patients with glioblastoma multiforme)11. Nevertheless, this route of administration has many obvious limitations. Currently available cannabis-based medicines are administered as capsules or using an oromucosal spray15. Preclinical animal models have yielded data indicating that systemic (oral or intraperitoneal) administration of cannabinoids effectively decreases tumour growth (GV, CS, and MG. Unpublished observations), and so it seems reasonable that future clinical studies with the goal of determining the efficacy of cannabinoids as antitumour agents use oral or oromucosal routes of administration.
Two currently ongoing clinical trials could shed some light on these issues. One of the studies is a phase i/iitrial aimed at evaluating the combined effect of Sativex (an oromucosal cannabis extract whose main active components are thc and cbd in a c. 1:1 ratio) and temozolomide in patients with recurrent glioblastoma multiforme (https://clinicaltrials.gov/ct2/show/NCT01812603 and https://clinicaltrials.gov/ct2/show/NCT01812616). The other is a phase ii trial aimed at evaluating the effect of cbd as single treatment in patients with solid tumours (https://clinicaltrials.gov/ct2/show/NCT02255292). Hopefully, in the near future, new clinical trials will start, helping to determine whether cannabinoids can be used, for other than their palliative effects, in the treatment of cancer patients
CONCLUSIONS AND FUTURE DIRECTIONS
It is widely believed that strategies aimed at reducing mortality from cancer should consist of targeted therapies capable of providing the most efficacious and selective treatment for each individual tumour and patient. Thus, the major focus of anticancer drug development has progressively moved from nonspecific chemotherapies to molecularly-targeted inhibitors. However, despite the huge amount of preclinical literature on how these rationally designed compounds work, their use in clinical practice is still limited.
How do cannabinoid-based medicines fit into this ongoing scenario? Consider glioma, the type of cancer in which the most detailed cannabinoid research has been conducted to date. As discussed here, engagement of a molecular target (the cb receptors) by a family of selective drugs (thc and other cannabinoid agonists) inhibits tumour growth in animal models through a well-established mechanism of action that also seems to operate in human patients. Moreover, cannabinoids potentiate the antitumour efficacy of temozolomide and alk inhibitors in mice harbouring gliomas. Those findings provide preclinical proof-of-concept that “cannabinoid sensitizers” could improve the clinical efficacy of classical cytotoxic drugs in glioblastoma (Figure 2) and perhaps other highly malignant tumours such as pancreatic cancer, melanoma, and hepatocellular carcinoma. However, further research is required to define the precise molecular cross-talk between cannabinoids and chemotherapeutic drugs and to optimize the pharmacology of preclinical cannabinoid-based combination therapies.
With respect to patient stratification, the particular individuals that are potentially responsive to cannabinoid administration should be unequivocally determined. To that end, high-throughput approaches should be implemented to find cannabinoid therapy–associated biomarkers in tumour biopsies or, ideally, in easily acquired fluids containing circulating cancer cells or enhanced levels of resistance factors that might have been released by cancer cells. Such biomarkers would conceivably relate to cannabinoid pharmacodynamics—namely, expression and activity of cannabinoid receptors and their downstream cell-death-inducing effectors. The approach would be analogous to the biochemical evaluation of estrogen and ErbB2 receptors, which respectively predict benefit from endocrine therapies and trastuzumab in breast cancer. Predictive markers to define the sensitivity of a particular tumour to cannabinoid-based therapies could also include the status of growth factors, such as mdk in gliomas, and their receptors and signalling partners.
To summarize, cannabinoids induce tumour cell death and inhibit tumour angiogenesis and invasion in animal models of cancer, and there are indications that they act similarly in patients with glioblastoma. Given that cannabinoids show an acceptable safety profile, clinical trials testing them as single drugs or, ideally, in combination therapies in glioblastoma and other types of cancer are both warranted and urgently needed.
Acknowledgments
Research by the authors and colleagues in their groups is supported by grants from the Spanish Ministry of Economy and Competitiveness and the Fondo Europeo de Desarrollo Regional (PI12/02248, PI15/0339, FR2009-0052, and IT2009-0053 to GV, and PI11/00295 to CS), Comunidad de Madrid (S2011/BMD-2308 to MG), GW Pharmaceuticals (to GV and CS), Fundación Mutua Madrileña (AP101042012 to GV), and Fundació La Marató de TV3 (20134031 to GV).
CONFLICT OF INTEREST DISCLOSURES
We have read and understood Current Oncology’s policy on disclosing conflicts of interest, and we declare the following interests: GW Pharmaceuticals and Cellmid fund part of the research conducted by our laboratory. Likewise, a portion of the data obtained by the authors concerning the antitumoural action of cannabinoids is included in three patent applications presented by GW Pharmaceuticals.
REFERENCES
1. Gaoni Y, Mechoulam R. Isolation, structure and partial synthesis of an active constituent of hashish. J Am Chem Soc. 1964;86:1646–7. doi: 10.1021/ja01062a046. [Cross Ref]
2. Pertwee RG. The diverse cb1 and cb2 receptor pharmacology of three plant cannabinoids: delta9-tetrahydrocannabinol, cannabidiol and delta9-tetrahydrocannabivarin. Br J Pharmacol. 2008;153:199–215. doi: 10.1038/sj.bjp.0707442. [PMC free article] [PubMed] [Cross Ref]
3. Devane WA, Hanus L, Breuer A, et al. Isolation and structure of a brain constituent that binds to the cannabinoid receptor. Science. 1992;258:1946–9. doi: 10.1126/science.1470919. [PubMed] [Cross Ref]
4. Mechoulam R, Ben-Shabat S, Hanus L, et al. Identification of an endogenous 2-monoglyceride, present in canine gut, that binds to cannabinoid receptors. Biochem Pharmacol. 1995;50:83–90. doi: 10.1016/0006-2952(95)00109-D. [PubMed] [Cross Ref]
5. Sugiura T, Kondo S, Sukagawa A, et al. 2-Arachidonoylglycerol: a possible endogenous cannabinoid receptor ligand in brain. Biochem Biophys Res Commun. 1995;215:89–97. doi: 10.1006/bbrc.1995.2437.[PubMed] [Cross Ref]
6. Pertwee RG, Howlett AC, Abood ME, et al. International Union of Basic and Clinical Pharmacology. LXXIX. Cannabinoid receptors and their ligands: beyond cb1 and cb2. Pharmacol Rev. 2010;62:588–631. doi: 10.1124/pr.110.003004. [PMC free article] [PubMed] [Cross Ref]
7. Matsuda LA, Lolait SJ, Brownstein MJ, Young AC, Bonner TI. Structure of a cannabinoid receptor and functional expression of the cloned cdna. Nature. 1990;346:561–4. doi: 10.1038/346561a0. [PubMed][Cross Ref]
8. Munro S, Thomas KL, Abu-Shaar M. Molecular characterization of a peripheral receptor for cannabinoids. Nature. 1993;365:61–5. doi: 10.1038/365061a0. [PubMed] [Cross Ref]
9. Fernandez-Ruiz J, Romero J, Velasco G, Tolon R, Ramos J, Guzman M. Cannabinoid cb2 receptor: a new target for controlling neural cell survival? Trends Pharmacol Sci. 2007;28:39–45. doi: 10.1016/j.tips.2006.11.001. [PubMed] [Cross Ref]
10. Atwood BK, Mackie K. cb2: a cannabinoid receptor with an identity crisis. Br J Pharmacol. 2010;160:467–79. doi: 10.1111/j.1476-5381.2010.00729.x. [PMC free article] [PubMed] [Cross Ref]
11. Guzman M, Duarte MJ, Blazquez C, et al. A pilot clinical study of delta9-tetrahydrocannabinol in patients with recurrent glioblastoma multiforme. Br J Cancer. 2006;95:197–203. doi: 10.1038/sj.bjc.6603236. [PMC free article] [PubMed] [Cross Ref]
12. Sarfaraz S, Adhami VM, Syed DN, Afaq F, Mukhtar H. Cannabinoids for cancer treatment: progress and promise. Cancer Res. 2008;68:339–42. doi: 10.1158/0008-5472.CAN-07-2785. [PubMed] [Cross Ref]
13. Katona I, Freund TF. Endocannabinoid signaling as a synaptic circuit breaker in neurological disease. Nat Med. 2008;14:923–30. doi: 10.1038/nm.f.1869. [PubMed] [Cross Ref]
14. Pacher P, Batkai S, Kunos G. The endocannabinoid system as an emerging target of pharmacotherapy. Pharmacol Rev. 2006;58:389–462. doi: 10.1124/pr.58.3.2. [PMC free article] [PubMed] [Cross Ref]
15. Pertwee RG. Emerging strategies for exploiting cannabinoid receptor agonists as medicines. Br J Pharmacol. 2009;156:397–411. doi: 10.1111/j.1476-5381.2008.00048.x. [PMC free article] [PubMed][Cross Ref]
16. Guzman M. Cannabinoids: potential anticancer agents. Nat Rev Cancer. 2003;3:745–55. doi: 10.1038/nrc1188. [PubMed] [Cross Ref]
17. Velasco G, Sanchez C, Guzman M. Towards the use of cannabinoids as antitumour agents. Nat Rev Cancer. 2012;12:436–44. doi: 10.1038/nrc3247. [PubMed] [Cross Ref]
18. Cudaback E, Marrs W, Moeller T, Stella N. The expression level of cb1 and cb2 receptors determines their efficacy at inducing apoptosis in astrocytomas. PloS One. 2010;5:e8702. doi: 10.1371/journal.pone.0008702. [PMC free article] [PubMed] [Cross Ref]
19. Hart S, Fischer OM, Ullrich A. Cannabinoids induce cancer cell proliferation via tumor necrosis factor alpha-converting enzyme (tace/adam17)–mediated transactivation of the epidermal growth factor receptor. Cancer Res. 2004;64:1943–50. doi: 10.1158/0008-5472.CAN-03-3720. [PubMed] [Cross Ref]
20. McKallip RJ, Nagarkatti M, Nagarkatti PS. Delta-9-tetrahydrocannabinol enhances breast cancer growth and metastasis by suppression of the antitumor immune response. J Immunol. 2005;174:3281–9. doi: 10.4049/jimmunol.174.6.3281. [PubMed] [Cross Ref]
21. Zhu LX, Sharma S, Stolina M, et al. Delta-9-tetrahydrocannabinol inhibits antitumour immunity by a cb2 receptor-mediated, cytokine-dependent pathway. J Immunol. 2000;165:373–80. doi: 10.4049/jimmunol.165.1.373. [PubMed] [Cross Ref]
22. Malfitano AM, Ciaglia E, Gangemi G, Gazzerro P, Laezza C, Bifulco M. Update on the endocannabinoid system as an anticancer target. Expert Opin Ther Targets. 2011;15:297–308. doi: 10.1517/14728222.2011.553606. [PubMed] [Cross Ref]
23. Sanchez C, de Ceballos ML, Gomez del Pulgar T, et al. Inhibition of glioma growth in vivo by selective activation of the cb2 cannabinoid receptor. Cancer Res. 2001;61:5784–9. [PubMed]
24. Caffarel MM, Sarrio D, Palacios J, Guzman M, Sanchez C. Delta9-tetrahydrocannabinol inhibits cell cycle progression in human breast cancer cells through Cdc2 regulation. Cancer Res. 2006;66:6615–21. doi: 10.1158/0008-5472.CAN-05-4566. [PubMed] [Cross Ref]
25. Nomura DK, Long JZ, Niessen S, Hoover HS, Ng SW, Cravatt BF. Monoacylglycerol lipase regulates a fatty acid network that promotes cancer pathogenesis. Cell. 2010;140:49–61. doi: 10.1016/j.cell.2009.11.027. [PMC free article] [PubMed] [Cross Ref]
26. Thors L, Bergh A, Persson E, et al. Fatty acid amide hydrolase in prostate cancer: association with disease severity and outcome, cb1 receptor expression and regulation by il-4. PLoS One. 2010;5:e12275. doi: 10.1371/journal.pone.0012275. [PMC free article] [PubMed] [Cross Ref]
27. Zheng D, Bode AM, Zhao Q, et al. The cannabinoid receptors are required for ultraviolet-induced inflammation and skin cancer development. Cancer Res. 2008;68:3992–8. doi: 10.1158/0008-5472.CAN-07-6594. [PMC free article] [PubMed] [Cross Ref]
28. Joosten M, Valk PJ, Jorda MA, et al. Leukemic predisposition of pSca-1/Cb2 transgenic mice. Exp Hematol. 2002;30:142–9. doi: 10.1016/S0301-472X(01)00779-2. [PubMed] [Cross Ref]
29. Perez-Gomez E, Andradas C, Blasco-Benito S, et al. Role of cannabinoid receptor cb2 in her2 pro-oncogenic signaling in breast cancer. J Natl Cancer Inst. 2015;107:djv077. doi: 10.1093/jnci/djv077.[PubMed] [Cross Ref]
30. Wang D, Wang H, Ning W, Backlund MG, Dey SK, DuBois RN. Loss of cannabinoid receptor 1 accelerates intestinal tumor growth. Cancer Res. 2008;68:6468–76. doi: 10.1158/0008-5472.CAN-08-0896. [PMC free article] [PubMed] [Cross Ref]
31. Izzo AA, Aviello G, Petrosino S, et al. Increased endocannabinoid levels reduce the development of precancerous lesions in the mouse colon. J Mol Med (Berl) 2008;86:89–98. doi: 10.1007/s00109-007-0248-4. [PMC free article] [PubMed] [Cross Ref]
32. Blazquez C, Gonzalez-Feria L, Alvarez L, Haro A, Casanova ML, Guzman M. Cannabinoids inhibit the vascular endothelial growth factor pathway in gliomas. Cancer Res. 2004;64:5617–23. doi: 10.1158/0008-5472.CAN-03-3927. [PubMed] [Cross Ref]
33. Galve-Roperh I, Sanchez C, Cortes ML, Gómez del Pulgar T, Izquierdo M, Guzman M. Antitumoral action of cannabinoids: involvement of sustained ceramide accumulation and extracellular signal-regulated kinase activation. Nat Med. 2000;6:313–19. doi: 10.1038/73171. [PubMed] [Cross Ref]
34. Gomez del Pulgar T, Velasco G, Sanchez C, Haro A, Guzman M. De novo–synthesized ceramide is involved in cannabinoid-induced apoptosis. Biochem J. 2002;363:183–8. doi: 10.1042/0264-6021:3630183. [PMC free article] [PubMed] [Cross Ref]
35. Carracedo A, Lorente M, Egia A, et al. The stress-regulated protein p8 mediates cannabinoid-induced apoptosis of tumor cells. Cancer Cell. 2006;9:301–12. doi: 10.1016/j.ccr.2006.03.005. [PubMed][Cross Ref]
36. Encinar JA, Mallo GV, Mizyrycki C, et al. Human p8 is a hmg-i/y–like protein with dna binding activity enhanced by phosphorylation. J Biol Chem. 2001;276:2742–51. doi: 10.1074/jbc.M008594200.[PubMed] [Cross Ref]
37. Salazar M, Carracedo A, Salanueva IJ, et al. Cannabinoid action induces autophagy-mediated cell death through stimulation of er stress in human glioma cells. J Clin Invest. 2009;119:1359–72. doi: 10.1172/JCI37948. [PMC free article] [PubMed] [Cross Ref]
38. Du K, Herzig S, Kulkarni RN, Montminy M. trb3: a tribbles homolog that inhibits Akt/pkb activation by insulin in liver. Science. 2003;300:1574–7. doi: 10.1126/science.1079817. [PubMed] [Cross Ref]
39. Verfaillie T, Salazar M, Velasco G, Agostinis P. Linking er stress to autophagy: potential implications for cancer therapy. Int J Cell Biol. 2010;2010:930509. doi: 10.1155/2010/930509. [PMC free article][PubMed] [Cross Ref]
40. Mizushima N, Levine B, Cuervo AM, Klionsky DJ. Autophagy fights disease through cellular self-digestion. Nature. 2008;451:1069–75. doi: 10.1038/nature06639. [PMC free article] [PubMed] [Cross Ref]
41. Eisenberg-Lerner A, Bialik S, Simon HU, Kimchi A. Life and death partners: apoptosis, autophagy and the cross-talk between them. Cell Death Differ. 2009;16:966–75. doi: 10.1038/cdd.2009.33. [PubMed][Cross Ref]
42. Galluzzi L, Pietrocola F, Bravo-San Pedro JM, et al. Autophagy in malignant transformation and cancer progression. EMBO J. 2015;34:856–80. doi: 10.15252/embj.201490784. [PMC free article] [PubMed][Cross Ref]
43. Vara D, Salazar M, Olea-Herrero N, Guzman M, Velasco G, Diaz-Laviada I. Antitumoral action of cannabinoids on hepatocellular carcinoma: role of ampk-dependent activation of autophagy. Cell Death Differ. 2011;18:1099–111. doi: 10.1038/cdd.2011.32. [PMC free article] [PubMed] [Cross Ref]
44. Armstrong JL, Hill DS, McKee CS, et al. Exploiting cannabinoid-induced cytotoxic autophagy to drive melanoma cell death. J Invest Dermatol. 2015;135:1629–37. doi: 10.1038/jid.2015.45. [PubMed][Cross Ref]
45. Ellert-Miklaszewska A, Kaminska B, Konarska L. Cannabinoids down-regulate pi3k/Akt and Erk signalling pathways and activate proapoptotic function of Bad protein. Cell Signal. 2005;17:25–37. doi: 10.1016/j.cellsig.2004.05.011. [PubMed] [Cross Ref]
46. Blazquez C, Carracedo A, Barrado L, et al. Cannabinoid receptors as novel targets for the treatment of melanoma. FASEB J. 2006;20:2633–5. doi: 10.1096/fj.06-6638fje. [PubMed] [Cross Ref]
47. Caffarel MM, Moreno-Bueno G, Cerutti C, et al. JunD is involved in the antiproliferative effect of delta9-tetrahydrocannabinol on human breast cancer cells. Oncogene. 2008;27:5033–44. doi: 10.1038/onc.2008.145. [PubMed] [Cross Ref]
48. Carracedo A, Gironella M, Lorente M, et al. Cannabinoids induce apoptosis of pancreatic tumor cells via endoplasmic reticulum stress-related genes. Cancer Res. 2006;66:6748–55. doi: 10.1158/0008-5472.CAN-06-0169. [PubMed] [Cross Ref]
49. Salazar M, Lorente M, Garcia-Taboada E, et al. The pseudokinase tribbles homologue-3 plays a crucial role in cannabinoid anticancer action. Biochim Biophys Acta. 2013;1831:1573–8. doi: 10.1016/j.bbalip.2013.03.014. [PubMed] [Cross Ref]
50. Caffarel MM, Andradas C, Perez-Gomez E, Guzman M, Sanchez C. Cannabinoids: a new hope for breast cancer therapy? Cancer Treat Rev. 2012;38:911–18. doi: 10.1016/j.ctrv.2012.06.005. [PubMed][Cross Ref]
51. Sarfaraz S, Afaq F, Adhami VM, Malik A, Mukhtar H. Cannabinoid receptor agonist-induced apoptosis of human prostate cancer cells LNCaP proceeds through sustained activation of erk1/2 leading to G1 cell cycle arrest. J Biol Chem. 2006;281:39480–91. doi: 10.1074/jbc.M603495200. [PubMed] [Cross Ref]
52. Guindon J, Hohmann AG. The endocannabinoid system and cancer: therapeutic implication. Br J Pharmacol. 2011;163:1447–63. doi: 10.1111/j.1476-5381.2011.01327.x. [PMC free article] [PubMed][Cross Ref]
53. Galve-Roperh I, Aguado T, Palazuelos J, Guzman M. Mechanisms of control of neuron survival by the endocannabinoid system. Curr Pharm Des. 2008;14:2279–88. doi: 10.2174/138161208785740117.[PubMed] [Cross Ref]
54. Casanova ML, Blazquez C, Martinez-Palacio J, et al. Inhibition of skin tumor growth and angiogenesis in vivo by activation of cannabinoid receptors. J Clin Invest. 2003;111:43–50. doi: 10.1172/JCI200316116.[PMC free article] [PubMed] [Cross Ref]
55. Chan PC, Sills RC, Braun AG, Haseman JK, Bucher JR. Toxicity and carcinogenicity of delta 9-tetrahydrocannabinol in Fischer rats and B6C3F1 mice. Fundam Appl Toxicol. 1996;30:109–17. doi: 10.1006/faat.1996.0048. [PubMed] [Cross Ref]
56. Lorente M, Torres S, Salazar M, et al. Stimulation of the midkine/alk axis renders glioma cells resistant to cannabinoid antitumoral action. Cell Death Differ. 2011;18:959–73. doi: 10.1038/cdd.2010.170.[PMC free article] [PubMed] [Cross Ref]
57. Ligresti A, Moriello AS, Starowicz K, et al. Antitumor activity of plant cannabinoids with emphasis on the effect of cannabidiol on human breast carcinoma. J Pharmacol Exp Ther. 2006;318:1375–87. doi: 10.1124/jpet.106.105247. [PubMed] [Cross Ref]
58. Massi P, Valenti M, Vaccani A, et al. 5-Lipoxygenase and anandamide hydrolase (faah) mediate the antitumor activity of cannabidiol, a non-psychoactive cannabinoid. J Neurochem. 2008;104:1091–100. doi: 10.1111/j.1471-4159.2007.05073.x. [PubMed] [Cross Ref]
59. Shrivastava A, Kuzontkoski PM, Groopman JE, Prasad A. Cannabidiol induces programmed cell death in breast cancer cells by coordinating the cross-talk between apoptosis and autophagy. Mol Cancer Ther. 2011;10:1161–72. doi: 10.1158/1535-7163.MCT-10-1100. [PubMed] [Cross Ref]
60. Nabissi M, Morelli MB, Santoni M, Santoni G. Triggering of the trpv2 channel by cannabidiol sensitizes glioblastoma cells to cytotoxic chemotherapeutic agents. Carcinogenesis. 2012;34:48–57. doi: 10.1093/carcin/bgs328. [PubMed] [Cross Ref]
61. Blazquez C, Casanova ML, Planas A, et al. Inhibition of tumor angiogenesis by cannabinoids. FASEB J. 2003;17:529–31. [PubMed]
62. Portella G, Laezza C, Laccetti P, De Petrocellis L, Di Marzo V, Bifulco M. Inhibitory effects of cannabinoid cb1 receptor stimulation on tumor growth and metastatic spreading: actions on signals involved in angiogenesis and metastasis. FASEB J. 2003;17:1771–3. [PubMed]
63. Pisanti S, Borselli C, Oliviero O, Laezza C, Gazzerro P, Bifulco M. Antiangiogenic activity of the endocannabinoid anandamide: correlation to its tumor-suppressor efficacy. J Cell Physiol. 2007;211:495–503. doi: 10.1002/jcp.20954. [PubMed] [Cross Ref]
64. Blazquez C, Salazar M, Carracedo A, et al. Cannabinoids inhibit glioma cell invasion by down-regulating matrix metalloproteinase-2 expression. Cancer Res. 2008;68:1945–52. doi: 10.1158/0008-5472.CAN-07-5176. [PubMed] [Cross Ref]
65. Grimaldi C, Pisanti S, Laezza C, et al. Anandamide inhibits adhesion and migration of breast cancer cells. Exp Cell Res. 2006;312:363–73. doi: 10.1016/j.yexcr.2005.10.024. [PubMed] [Cross Ref]
66. Qamri Z, Preet A, Nasser MW, et al. Synthetic cannabinoid receptor agonists inhibit tumor growth and metastasis of breast cancer. Mol Cancer Ther. 2009;8:3117–29. doi: 10.1158/1535-7163.MCT-09-0448.[PMC free article] [PubMed] [Cross Ref]
67. Preet A, Ganju RK, Groopman JE. Delta9-tetrahydrocannabinol inhibits epithelial growth factor-induced lung cancer cell migration in vitro as well as its growth and metastasis in vivo. Oncogene. 2008;27:339–46. doi: 10.1038/sj.onc.1210641. [PubMed] [Cross Ref]
68. Ramer R, Hinz B. Inhibition of cancer cell invasion by cannabinoids via increased expression of tissue inhibitor of matrix metalloproteinases-1. J Natl Cancer Inst. 2008;100:59–69. doi: 10.1093/jnci/djm268.[PubMed] [Cross Ref]
69. McAllister SD, Murase R, Christian RT, et al. Pathways mediating the effects of cannabidiol on the reduction of breast cancer cell proliferation, invasion, and metastasis. Breast Cancer Res Treat. 2011;129:37–47. doi: 10.1007/s10549-010-1177-4. [PMC free article] [PubMed] [Cross Ref]
70. Soroceanu L, Murase R, Limbad C, et al. Id-1 is a key transcriptional regulator of glioblastoma aggressiveness and a novel therapeutic target. Cancer Res. 2012;73:1559–69. doi: 10.1158/0008-5472.CAN-12-1943. [PMC free article] [PubMed] [Cross Ref]
71. Newton CA, Chou PJ, Perkins I, Klein TW. cb1 and cb2 cannabinoid receptors mediate different aspects of delta-9-tetrahydrocannabinol (thc)–induced T helper cell shift following immune activation by Legionella pneumophila infection. J Neuroimmune Pharmacol. 2009;4:92–102. doi: 10.1007/s11481-008-9126-2. [PubMed] [Cross Ref]
72. Lu T, Newton C, Perkins I, Friedman H, Klein TW. Cannabinoid treatment suppresses the T-helper cell-polarizing function of mouse dendritic cells stimulated with Legionella pneumophila infection. J Pharmacol Exp Ther. 2006;319:269–76. doi: 10.1124/jpet.106.108381. [PubMed] [Cross Ref]
73. Steffens S, Veillard NR, Arnaud C, et al. Low dose oral cannabinoid therapy reduces progression of atherosclerosis in mice. Nature. 2005;434:782–6. doi: 10.1038/nature03389. [Erratum in: Nature 2005;435:528] [PubMed] [Cross Ref]
74. Hegde VL, Nagarkatti M, Nagarkatti PS. Cannabinoid receptor activation leads to massive mobilization of myeloid-derived suppressor cells with potent immunosuppressive properties. Eur J Immunol. 2010;40:3358–71. doi: 10.1002/eji.201040667. [PMC free article] [PubMed] [Cross Ref]
75. Burstein SH, Zurier RB. Cannabinoids, endocannabinoids, and related analogs in inflammation. AAPS J. 2009;11:109–19. doi: 10.1208/s12248-009-9084-5. [PMC free article] [PubMed] [Cross Ref]
76. Liu WM, Fowler DW, Dalgleish AG. Cannabis-derived substances in cancer therapy—an emerging anti-inflammatory role for the cannabinoids. Curr Clin Pharmacol. 2010;5:281–7. doi: 10.2174/157488410793352049. [PubMed] [Cross Ref]
77. Mirkin BL, Clark S, Zheng X, et al. Identification of midkine as a mediator for intercellular transfer of drug resistance. Oncogene. 2005;24:4965–74. doi: 10.1038/sj.onc.1208671. [PubMed] [Cross Ref]
78. Kadomatsu K. The midkine family in cancer, inflammation and neural development. Nagoya J Med Sci. 2005;67:71–82. [PubMed]
79. Palmer RH, Vernersson E, Grabbe C, Hallberg B. Anaplastic lymphoma kinase: signalling in development and disease. Biochem J. 2009;420:345–61. doi: 10.1042/BJ20090387. [PMC free article][PubMed] [Cross Ref]
80. Lonardi S, Tosoni A, Brandes AA. Adjuvant chemotherapy in the treatment of high grade gliomas. Cancer Treat Rev. 2005;31:79–89. doi: 10.1016/j.ctrv.2004.12.005. [PubMed] [Cross Ref]
81. Nieder C, Adam M, Molls M, Grosu AL. Therapeutic options for recurrent high-grade glioma in adult patients: recent advances. Crit Rev Oncol Hematol. 2006;60:181–93. doi: 10.1016/j.critrevonc.2006.06.007. [PubMed] [Cross Ref]
82. Purow B, Schiff D. Advances in the genetics of glioblastoma: are we reaching critical mass? Nat Rev Neurol. 2009;5:419–26. doi: 10.1038/nrneurol.2009.96. [PMC free article] [PubMed] [Cross Ref]
83. Lorente M, Carracedo A, Torres S, et al. Amphiregulin is a factor for resistance of glioma cells to cannabinoid-induced apoptosis. Glia. 2009;57:1374–85. doi: 10.1002/glia.20856. [PubMed] [Cross Ref]
84. Stupp R, Mason WP, van den Bent MJ, et al. Radiotherapy plus concomitant and adjuvant temozolomide for glioblastoma. N Engl J Med. 2005;352:987–96. doi: 10.1056/NEJMoa043330. [PubMed][Cross Ref]
85. Torres S, Lorente M, Rodriguez-Fornes F, et al. A combined preclinical therapy of cannabinoids and temozolomide against glioma. Mol Cancer Ther. 2011;10:90–103. doi: 10.1158/1535-7163.MCT-10-0688.[PubMed] [Cross Ref]
86. de Bono JS, Ashworth A. Translating cancer research into targeted therapeutics. Nature. 2010;467:543–9. doi: 10.1038/nature09339. [PubMed] [Cross Ref]
87. Grande E, Bolos MV, Arriola E. Targeting oncogenic alk: a promising strategy for cancer treatment. Mol Cancer Ther. 2011;10:569–79. doi: 10.1158/1535-7163.MCT-10-0615. [PubMed] [Cross Ref]
88. Donadelli M, Dando I, Zaniboni T, et al. Gemcitabine/cannabinoid combination triggers autophagy in pancreatic cancer cells through a ros-mediated mechanism. Cell Death Dis. 2011;2:e152. doi: 10.1038/cddis.2011.36. [PMC free article] [PubMed] [Cross Ref]
89. Miyato H, Kitayama J, Yamashita H, et al. Pharmacological synergism between cannabinoids and paclitaxel in gastric cancer cell lines. J Surg Res. 2009;155:40–7. doi: 10.1016/j.jss.2008.06.045. [PubMed][Cross Ref]
90. Gustafsson SB, Lindgren T, Jonsson M, Jacobsson SO. Cannabinoid receptor–independent cytotoxic effects of cannabinoids in human colorectal carcinoma cells: synergism with 5-fluorouracil. Cancer Chemother Pharmacol. 2009;63:691–701. doi: 10.1007/s00280-008-0788-5. [PubMed] [Cross Ref]
91. Massi P, Vaccani A, Bianchessi S, Costa B, Macchi P, Parolaro D. The non-psychoactive cannabidiol triggers caspase activation and oxidative stress in human glioma cells. Cell Mol Life Sci. 2006;63:2057–66. doi: 10.1007/s00018-006-6156-x. [PubMed] [Cross Ref]
92. McAllister SD, Christian RT, Horowitz MP, Garcia A, Desprez PY. Cannabidiol as a novel inhibitor of Id-1 gene expression in aggressive breast cancer cells. Mol Cancer Ther. 2007;6:2921–7. doi: 10.1158/1535-7163.MCT-07-0371. [PubMed] [Cross Ref]
93. Marcu JP, Christian RT, Lau D, et al. Cannabidiol enhances the inhibitory effects of delta9-tetrahydrocannabinol on human glioblastoma cell proliferation and survival. Mol Cancer Ther. 2010;9:180–9. doi: 10.1158/1535-7163.MCT-09-0407. [PMC free article] [PubMed] [Cross Ref]
94. Scott KA, Dalgleish AG, Liu WM. The combination of cannabidiol and Delta9-tetrahydrocannabinol enhances the anticancer effects of radiation in an orthotopic murine glioma model. Mol Cancer Ther. 2014;13:2955–67. doi: 10.1158/1535-7163.MCT-14-0402. [PubMed] [Cross Ref]
95. Bifulco M, Laezza C, Portella G, et al. Control by the endogenous cannabinoid system of ras oncogene-dependent tumor growth. FASEB J. 2001;15:2745–7. [PubMed]
96. Ligresti A, Bisogno T, Matias I, et al. Possible endocannabinoid control of colorectal cancer growth. Gastroenterology. 2003;125:677–87. doi: 10.1016/S0016-5085(03)00881-3. [PubMed] [Cross Ref]
Curr Oncol. 2016 Mar; 23(Suppl 2): S23–S32.
Published online 2016 Mar 16. doi: 10.3747/co.23.3080
G. Velasco, PhD,*†‡ C. Sánchez, PhD,*§ and M. Guzmán, PhD*†‖
Abstract
In addition to the well-known palliative effects of cannabinoids on some cancer-associated symptoms, a large body of evidence shows that these molecules can decrease tumour growth in animal models of cancer. They do so by modulating key cell signalling pathways involved in the control of cancer cell proliferation and survival. In addition, cannabinoids inhibit angiogenesis and decrease metastasis in various tumour types in laboratory animals. In this review, we discuss the current understanding of cannabinoids as antitumour agents, focusing on recent discoveries about their molecular mechanisms of action, including resistance mechanisms and opportunities for their use in combination therapy. Those observations have already contributed to the foundation for the development of the first clinical studies that will analyze the safety and potential clinical benefit of cannabinoids as anticancer agents.
Keywords: Cannabinoids, apoptosis, autophagy, cell proliferation, angiogenesis, cell signalling, combination therapy
INTRODUCTION
Preparations of Cannabis sativa L. (marijuana) have been used for many centuries both medicinally and recreationally. However, the chemical structures of their unique active components—the cannabinoids—were not elucidated until the 1960s. Three decades later, the first solid clues to cannabinoid molecular action were established, which led to an impressive expansion of basic cannabinoid research and a renaissance in the study of the therapeutic effects of cannabinoids in various fields, including oncology. Today, it is widely accepted that, of the approximately 108 cannabinoids produced by C. sativa, Δ9-tetrahydrocannabinol (thc) is the most relevant because of its high potency and abundance in plant preparations1,2.
Tetrahydrocannabinol exerts a wide variety of biologic effects by mimicking endogenous substances—the endocannabinoids anandamide3 and 2-arachidonoylglycerol4,5—that engage specific cell-surface cannabinoid receptors6. So far, two major cannabinoid-specific receptors—cb1 and cb2—have been cloned from mammalian tissues and characterized7,8. In addition, other receptors such as the transient receptor potential cation channel subfamily V, member 1, and the orphan G protein–coupled receptor 55 have been proposed to act as endocannabinoid receptors6. Most of the effects produced by cannabinoids in the nervous system and in non-neural tissues rely on cb1 receptor activation. In contrast, the cb2 receptor was initially described to be present in the immune system6, but was more recently shown to also be expressed in cells from other origins9,10. Notably, expression of the cb1 and cb2 receptors has been found in many types of cancer cells, but not necessarily correlating with the expression of those receptors in the tissue of origin9,11,12.
The endocannabinoids, together with their receptors and the proteins responsible for their synthesis, transport, and degradation, constitute the endocannabinoid system. Aside from its pivotal neuromodulatory activity13, the endocannabinoid system exerts other regulatory functions in the body such as control of cardiovascular tone, energy metabolism, immunity, and reproduction14,15. This miscellaneous activity makes the pharmacologic manipulation of the endocannabinoid system a promising strategy for the management of many diseases. Specifically, cannabinoids are well known to exert palliative effects in cancer patients14,15. Their best-established use is the inhibition of chemotherapy-induced nausea and vomiting15,16. Today, capsules of thc (Marinol: AbbVie, North Chicago, IL, U.S.A.) and its synthetic analogue nabilone (Cesamet: Meda Pharmaceuticals, Somerset, NJ, U.S.A.) are approved for that purpose. Cannabinoids also inhibit pain, and thus a standardized cannabis extract (Sativex: GW Pharmaceuticals, Salisbury, U.K.) has already been approved in Canada and is currently the subject of large-scale phase iii clinical trials for managing cancer-associated pain. Another potential palliative effect of cannabinoids in oncology, supported by phase iii clinical trials, includes appetite stimulation and attenuation of wasting. In that respect, Marinol can currently be prescribed for anorexia associated with weight loss in aids patients.
The therapeutic potential of cannabinoids in oncology might not be restricted to their aforementioned palliative actions. Thus, numerous studies have provided evidence that thc and other cannabinoids exhibit antitumour effects in a wide array of animal models of cancer12,16,17. Moreover, those observations led to the development of several clinical studies to investigate the antitumour activity of thc in humans (see “Clinical Antitumour Effects of Cannabinoids” later in this article). Nonetheless, a few studies have shown that, under certain conditions, cannabinoid treatment can stimulate cancer cell proliferation in vitro18,19 and interfere with the tumour-suppressor role of the immune system20,21. Likewise, reports about the role of the endocannabinoid system in cancer (tumour-suppressor or oncogenic) are conflicting22.
Reports concerning the biologic role of the endocannabinoid system in cancer physiopathology are sparse. Although some exceptions that might be tumour-type-specific are known, cannabinoid receptors and their endogenous ligands are both generally upregulated in tumour tissue compared with non-tumour tissue16,22–24. Additionally, various studies have associated the expression levels of cannabinoid receptors, endocannabinoids, or endocannabinoid-metabolizing enzymes with tumour aggressiveness22,25,26, which suggests that the endocannabinoid system might be overactivated in cancer and hence pro-tumourigenic22. In support of that hypothesis, reports showed that genetic ablation of cb1 and cb2 receptors in mouse models of cancer reduces ultraviolet light–induced skin carcinogenesis27, that overexpression of cb2 receptor enhances the predisposition to leukemia after leukemia viral infection28, and that cb2 promotes her2 (human epidermal growth factor receptor 2) pro-oncogenic signalling in breast cancer29.
Conversely, and in line with the evidence supporting the hypothesis that pharmacologic activation of cannabinoid receptors decreases tumour growth12,16, upregulation of endocannabinoid-degrading enzymes has been observed in aggressive human tumours and cancer cell lines25,26, indicating that endocannabinoid signalling can also have a tumour-suppressive role. In support of that observation, deletion of cb1 receptors was noted to accelerate intestinal tumour growth in a genetic mouse model of colon cancer30, increased endocannabinoid levels were observed to diminish azoxymethane-induced precancerous lesions in the mouse colon31, and reduction in the expression of the endocannabinoid-degrading enzyme monoacylglycerol lipase was seen to decrease tumour growth in xenografted mice25.
Further studies—including analyses of the activation of the precise signalling mechanisms involved in the regulation of cannabinoid-induced cell death, and of cell proliferation upon genetic or pharmacologic manipulation of the endocannabinoid system—are therefore needed to clarify the contextual determinants that result in the system acting as either a guardian or an inducer of tumourigenesis or tumour progression. The present review summarizes such observations and provides an integrated view of the molecular mechanisms responsible for cannabinoid antitumour activity. It also discusses the experimental evidence supporting the existence of mechanisms of resistance to the cell death–promoting actions of thc in certain types of cancer cells, the strategies that could possibly be used to overcome such resistance, and the preclinical data supporting the potential usefulness of the combined administration of cannabinoids and other drugs in anticancer therapies.
PRECLINICAL ANTITUMOUR ACTIVITY
Since the late 1990s, a large body of evidence has accumulated demonstrating that various cannabinoids exert antitumour effects in a wide variety of experimental models of cancer, ranging from cancer cell lines in culture to genetically-engineered mice (reviewed by Velasco et al.17). Multiple cannabinoids have shown this activity, including thc; the endocannabinoids 2-arachidonoylglycerol and anandamide; and various synthetic cannabinoid receptor agonists that have either comparable affinity for the cb1 and cb2 receptors (for example, WIN 55,212-2 or HU-210), a higher affinity for cb1 (for example, methanandamide), or a higher affinity for cb2 (for example, JWH-133). Those findings strongly support that, aside from the role played by the endogenous cannabinoid system in cancer, pharmacologic stimulation of cb receptors is, in most cases, antitumourigenic. Nonetheless, a few reports have proposed a tumour-promoting effect of cannabinoids18–21. Those apparently conflicting observations are discussed in the next subsection.
Mechanisms of Antitumour Effects
Cannabinoids impair tumour progression at various levels. Their most prevalent effect is the induction of cancer cell death by apoptosis and the inhibition of cancer cell proliferation. At least one of those actions has been demonstrated in almost all cancer cell types tested17. In addition, in vivo experiments have shown that cannabinoids impair tumour angiogenesis and block invasion and metastasis.
Induction of Cancer Cell Death
A significant amount of the research conducted so far on the mechanism of cannabinoid antitumour activity has focussed on glioma cells. Initial studies showed that thc and other cannabinoids induce the apoptotic death of glioma cells by cb1- and cb2-dependent stimulation of the de novo synthesis of the pro-apoptotic sphingolipid ceramide23,32–34. Further studies based on the analysis of the gene expression profile of thc-sensitive and -resistant glioma cells yielded further insight into the specific signalling events downstream of ceramide that are activated in cancer cells by cannabinoids35. Thus, it was found that treatment with thc results in enhanced expression of the stress-regulated protein p8 (nupr1), a transcriptional regulator that has been implicated in the control of tumourigenesis and tumour progression36, together with several of its downstream targets, such as the endoplasmic reticulum (er) stress–related transcription factors atf4 and chop, and the pseudokinase tribbles homologue 3 (trib3)35. This thc-triggered stimulation of the p8-regulated pathway (Figure 1) enhances the inhibitory interaction of trib3 with a pro-survival kinase, akt37,38, which leads to inhibition of the mammalian target of rapamycin complex 1 (mtorc1) and the subsequent stimulation of autophagy-mediated cell death37.
Cannabinoid-induced apoptosis relies on the stimulation of endoplasmic reticular (ER) stress and autophagy. Here, the mechanism of cannabinoid-induced apoptosis in glioma, pancreatic, and hepatocellular carcinoma cells is depicted. This signalling route could constitute the main mechanism of cannabinoid-induced cell death, with some variations inherent to different types of cancer cells. CB1 = cannabinoid CB1 receptor; CB2 = cannabinoid CB2 receptor; SPT = serine palmitoyltransferase; elF2α = eukaryotic translation initiation factor 2α; P inscribed in a circle = protein phosphorylation upon treatment with Δ9-tetrahydrocannabinol (THC); ATF-4 = activating transcription factor 4; CHOP = C/EBP homologous protein; AKT = protein kinase B; TRIB3 = tribbles-homologue 3; mTORC2 = mammalian target of rapamycin complex 2; CDKs = cyclin-dependent kinases; pRb = retinoblastoma protein; CaCMKKβ = calcium/calmodulin–dependent protein kinase kinase 2β; AMPK = AMP-activated protein kinase.
Autophagy is an essential cellular process participating in a number of physiologic functions within the cell39,40. During autophagy, organelles and other cytoplasmic components are engulfed within double-membrane vesicles called “autophagosomes.” The maturation of those vesicles involves their fusion with lysosomes, which leads in turn to the degradation of the autophagosome components by lysosomal enzymes40. Autophagy is primarily a cytoprotective mechanism, although its activation can also lead to cell death40–42.
Cannabinoids induce autophagy in various types of cancer cells in culture, and pharmacologic or genetic inhibition of autophagy prevents cannabinoid antitumour action in various animal models of cancer (Figure 1), thus demonstrating that autophagy is important for cannabinoid antineoplastic activity37,43,44. Moreover, autophagy blockade prevents cannabinoid-induced apoptosis and cell death, whereas apoptosis blockade prevents cannabinoid-induced cell death, but not autophagy37,43,44. Those observations indicate that autophagy is upstream of apoptosis in the mechanism of cannabinoid-induced cell death (Figure 1).
The importance of this pathway is highlighted by the ability of various chemical and genetic manipulations to block cannabinoid-induced cell death. In hepatocellular carcinoma cells, the cannabinoid-evoked and erstress–dependent activation of calcium/calmodulin-dependent protein kinase kinase 2β and amp-activated protein kinase leads, together with the p8–trib3 pathway, to autophagy and apoptosis43. The cannabinoid-evoked inhibition of akt was able to promote cycle arrest in breast cancer and melanoma cells, as well as apoptosis through additional mechanisms, including decreased phosphorylation of the pro-apoptotic protein bcl2-associated agonist of cell death45 and activation of the cyclin-dependent kinase inhibitory proteins p21 and p2724,46,47, leading to the subsequent decreased phosphorylation of the retinoblastoma protein, which would thus arrest the cell cycle.
The direct participation of the p8/trib3–mediated autophagy pathway in the antitumour action of cannabinoids has been clearly demonstrated in glioma cells, pancreatic and hepatic cancer cells, and melanoma cells35,37,43,44,48,49. At least part of that signalling route has also been found to be upregulated with cannabinoid treatment in other types of cancer cells, an observation which suggests that—with some variations—this pathway could be a general mechanism by which activation of cb1 and cb2 receptors promotes cancer cell death.
Additional mechanisms might nonetheless cooperate with the p8/trib3–mediated autophagy pathway to evoke cancer cell death (Figure 1). For example, in hepatocellular carcinoma cells, thc and the cb2 receptor agonist JWH-015 can trigger an er stress–dependent activation of amp-activated protein kinase that cooperates with the trib3-mediated inhibition of the akt–mtorc1 axis in the stimulation of autophagy-mediated cell death44. In melanoma46, breast carcinoma24,50, and prostate carcinoma51 cells, cannabinoids can induce cell-cycle arrest in concert with apoptosis24,46,51. Notably, cannabinoid antiproliferative action—at least in melanoma46 and breast cancer24 cells—also relies on akt inhibition.
Likewise, the effect of cannabinoids in hormone- dependent tumours might rely, at least in part, on the ability to interfere with the activation of growth factor receptors12,16. Some of those and other mechanisms52 might participate, together with the autophagy-mediated cell death pathway, in the cytotoxic action of cannabinoids in various types of cancer cells. However, further investigation is required to clarify the issue.
Research conducted during the last few years has shed light on the intracellular signalling mechanisms underlying cannabinoid anticancer action. However, a number of important observations—in particular, those related to the role played by cannabinoid receptors in the triggering of the signals—remain to be clarified. For example, in contrast to the death-promoting action of cannabinoids on cancer cells, the viability of normal (non-transformed) cells is unaffected or, under certain conditions, even enhanced by cannabinoid challenge33–35,37,53. For example, thc treatment of astrocytes (a cell type that expresses functional cb1 receptors) does not trigger the activation of er stress, upregulation of the p8 pathway, inhibition of the akt–mtorc1 axis, or stimulation of autophagy and apoptosis, even when concentrations of thc higher than those that promote glioma cell death are used35,37. Similar results were obtained for primary embryonic fibroblasts35,41 and other types of non-transformed cells expressing functional cannabinoid receptors in comparison with their transformed counterparts24,46,54,55. Thus, stimulation of cannabinoid receptors seems to be coupled with the activation of different signalling mechanisms in transformed and non-transformed cells. The precise molecular reasons for this variation in behaviour remain as an important open question in the cannabinoid field. Another intriguing observation is that, in some types of cancer cells (such as glioma cells), pharmacologic blockade of either cb1 or cb2 prevents cannabinoid-induced cell death with similar efficacy33,56, and yet in other types of cancer cells (pancreatic48, breast24, or hepatic43 carcinoma cells, for example), antagonists of cb2 but not of cb1 inhibit cannabinoid antitumour actions. The reason that cannabinoids produce their antitumour actions through one or the other of these receptor types depending on the type of cancer cell has yet to be established.
Some cannabinoid receptor agonists promote cancer cell death more efficiently than other agonists that exhibit similar or even higher affinity for the cb1 or cb2 receptors. For example, thc promotes cancer cell death in a cb1- or cb2-dependent manner (or both) at lower concentrations than does the synthetic cannabinoid receptor agonist WIN 55,212-2, although the latter agent shows significantly higher affinity for cb1 and cb2 in binding assays6.
Further work aimed at investigating, for example, cb receptor homo- or hetero-oligomerization in response to various cannabinoid agonists, their associations with specific domains in the plasma membrane such as lipid rafts, changes in the subcellular location of cb receptors, and the selective coupling to various G proteins and other signalling proteins, will be essential to answer the foregoing questions and to precisely define the role played by each cannabinoid receptor type as an anticancer signalling platform.
Notably, cannabidiol (cbd), a phytocannabinoid with a low affinity for cannabinoid receptors15, and other marijuana-derived cannabinoids57 have also been proposed to promote the apoptotic death of cancer cells acting independently of the cb1 and cb2 receptors. The mechanism by which cbd produces this effect has not as yet been completely clarified, but it seems to rely—at least in part—on its ability to enhance the production of reactive oxygen species in cancer cells58,59. It has also been proposed that cbd might activate trpv2 receptors to promote glioma cell death60.
Inhibition of Angiogenesis, Invasion, and Metastasis
In cancer cells, cannabinoids block the activation of the vascular endothelial growth factor (vegf) pathway, an inducer of angiogenesis. Specifically, various elements of the cascade, such as the main ligand (vegf) and the active forms of its main receptors (vegfr1 and vegfr2), are downregulated with cannabinoid treatment of skin carcinomas54, gliomas32,61, and thyroid carcinomas62. In vascular endothelial cells, cannabinoid receptor activation inhibits proliferation and migration, and induces apoptosis61,63. Those and perhaps other cannabinoid-evoked actions result in a normalized tumour vasculature—that is, smaller and fewer vessels that are more differentiated and less leaky.
Likewise, cb1 or cb2 receptor agonists (or both) reduce the formation of distant tumour masses in animal models of both induced and spontaneous metastasis, and inhibit adhesion, migration, and invasiveness of glioma64, breast65,66, lung67,68, and cervical68 cancer cells in culture. Those effects depend, at least in part, on the modulation of extracellular proteases (such as matrix metalloproteinase 2)64 and their inhibitors (such as tissue inhibitor of matrix metalloproteinases 1)68.
Notably, pharmacologic inhibition of ceramide biosynthesis abrogates the antitumour and antiangiogenic effect of cb1 or cb2 receptor agonists (or both) in glioma xenografts, and decreases vegf production by glioma cells in vitro and in vivo32. Likewise, inhibition of matrix metalloproteinase 2 expression and glioma cell invasion is prevented by blocking ceramide biosynthesis and by knocking down p8 expression64. Although further research is still necessary to precisely define the molecular mechanisms responsible for those actions of cannabinoids, the observations indicate that the ceramide/p8–regulated pathway plays a general role in the antitumour activity of cannabinoids targeting cb1 and cb2.
It is worth noting that cbd, by acting independently of the cb1 and cb2 receptors, produces a remarkable anti-tumour effect—including reduction of invasiveness and metastasis—in various animal models of cancer. This effect of cbd seems to rely, at least in part, on the downregulation of the helix-loop-helix transcription factor inhibitor of dna binding 169,70.
Regulation of Antitumour Immunity
Notably, stimulation of cannabinoid receptors can lead to important changes in the processes that regulate anti-tumour immunity. Thus, for example, treatment of mice with thc triggers a shift (from Th1 to Th2) of cytokine profile20,71–73 and induces mobilization of myeloid-derived suppressor cells74, two events that play a critical role in the suppression of antitumour immunity. In agreement with that notion, stimulation of cb2 has been proposed in some reports to enhance tumourigenesis by interfering with tumour surveillance by the immune system20,21.
By contrast, cannabinoids can also enhance immune system–mediated tumour surveillance in some contexts: the antitumour action of WIN 55,212-2 (a mixed cb1 or cb2 agonist) or JWH-133 (a cb2-selective agonist) was more pronounced in melanoma xenografts generated in immunocompetent mice than in those generated in immunodeficient mice46, a finding which also indicates that, at least in this model, stimulation of cb2 inhibits tumour growth primarily through direct effects on cancer cells rather than necessarily by interfering with the normal antitumour function of the immune system. In line with that idea, treatment of immunocompetent rats with very high doses of thc (50 mg/kg daily 5 times per week) for 2 years lowered the incidence of several types of tumours and enhanced the overall survival of the animals55. Those observations might be related to the ability of thc to reduce inflammation75,76, an effect that might prevent certain types of cancer76,77.
For cannabinoid use to be clinically successful, anti-tumour effects will have to overcome immunosuppressive (potentially tumour-promoting) effects. Additional studies should clarify the issue. For example, it could be conceivable to study the effect of cannabinoid administration on the generation and progression of tumours with varying sensitivity to cannabinoids and generated in immunocompetent or immunodeficient mice in which the expression of cb1 or cb2 receptors (or both) in cells from the immune system has been genetically manipulated.
RESISTANCE MECHANISMS
Numerous studies have contributed to an appreciation of the heterogeneity of cancer, whereby each subtype of cancer—and even each individual tumour—exhibits a series of molecular characteristics that determines its behaviour and, in particular, its responsiveness to various anticancer drugs. In agreement with that line of reasoning, a recent report investigated the molecular features associated with the resistance of a collection of human glioma cell lines and primary cultures to cannabinoid antitumour action56. The study showed that, although the apoptotic effect of thc on glioma cells relied on the stimulation of cannabinoid receptors and activation of the p8-mediated autophagy pathway, the differences in the sensitivity to thc-induced cell death correlated with enhanced expression of a particular set of genes in the thc-resistant glioma cells rather than with the presence of different expression levels of cb1 or cb2 receptors56. Interestingly, upregulation of one of those genes, midkine (MDK), which encodes a growth factor that was previously associated with increased malignancy and resistance to anticancer therapies in several types of tumours77,78, correlates with lower overall survival in patients with glioblastoma56. Moreover, mdk plays a direct role in the resistance to thc action through stimulation of anaplastic lymphoma kinase (alk79). Thus, the stimulation of alk by mdk inhibits the thc-evoked autophagy-mediated cell-death pathway. Further research should clarify whether that mechanism could also be responsible for the resistance to other therapies of cancer cells expressing high levels of mdk. Interestingly, in vivo silencing of MDK or pharmacologic inhibition of alk in a mouse xenograft model abolishes the resistance to thc treatment of established tumours derived from cannabinoid-resistant glioma cells56.
Taken together, the foregoing findings support the idea that stimulation of the mdk–alk axis promotes resistance to thc antitumour action in gliomas and could help to set a foundation for the potential clinical use of thc in combination with inhibitors of the mdk–alk axis (Figure 2). Glioblastoma is highly resistant to current anticancer therapies80–82. Specifically, resistance of glioma cells to cannabinoid-induced cell death relies, at least in part, on enhanced expression of mdk and the subsequent activation of alk56. Likewise, enhanced expression of the heparin-bound epidermal growth factor receptor (egfr) ligand amphiregulin can promote resistance to thc antitumour action by stimulation of extracellular signal-regulated kinase (erk)83. The combination of thc with pharmacologic inhibitors of alk (or genetic inhibition of MDK) enhances cannabinoid action in resistant tumours, which provides a rationale for the design of targeted therapies capable of increasing cannabinoid antineoplastic activity56. Combinations of cannabinoids with classical chemotherapeutic drugs such as the alkylating agent temozolomide (the benchmark agent for the management of glioblastoma80,84) have been shown to produce a strong anticancer action in animal models85. Combining cannabinoids and temozolomide is thus a very attractive possibility for clinical studies aimed at investigating cannabinoid antitumour effects in glioblastoma. Other potentially interesting strategies to enhance cannabinoid anticancer action (still requiring additional experimental support from data obtained using preclinical models) could be to combine cannabinoids with er stress or autophagy inducers (or both) or with inhibitors of the mtorc1 axis.
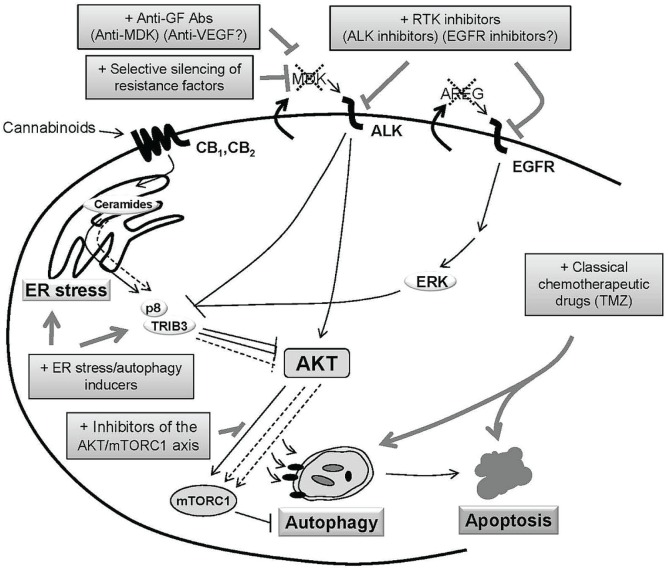
Possible strategies for optimizing cannabinoid-based therapies against gliomas. GF = growth factors; Abs = antibodies; MDK = growth factor midkine; VEGF = vascular endothelial growth factor; RTK = receptor tyrosine kinase; ALK = anaplastic lymphoma receptor tyrosine kinase; EGFR = epidermal growth factor receptor; CB1 = cannabinoid CB1 receptor; CB2 = cannabinoid CB2 receptor; AREG = amphiregulin; ER stress = endoplasmic reticular stress; ERK = extracellular signal-regulated kinase; TMZ = temozolomide; TRIB3 = tribbles-homologue 3; AKT = protein kinase B; mTORC1 = mammalian target of rapamycin complex 1.
In line with that idea, alk inhibitors have started to be used in clinical trials for the management of non-small-cell lung cancer and other types of tumours86,87. Future research should clarify whether this mechanism of resistance to cannabinoid action operates in other types of tumours. In agreement with that possibility, MDK silencing enhanced the sensitivity of cannabinoid-resistant pancreatic cancer cells to thc-induced cell death56.
The release by cancer cells of other growth factors has also been implicated in the mechanism of resistance to cannabinoid antitumour action. Thus, increased expression of amphiregulin is associated with enhanced resistance to thc antitumour action in glioma xenografts83. Notably illustrating how the dose of cannabinoids could be crucial for optimal therapeutic effect, low (submicromolar) concentrations of thc or other synthetic cannabinoid agonists enhance the proliferation of several cancer cell lines in vitro. That effect relies on activation of the protease adam17, the shedding of heparin-bound egfr ligands including amphiregulin and the subsequent stimulation of the erk and akt pathways19. In line with that idea, a recent report showed that treatment with the synthetic cannabinoid CP 55,940 increases the proliferation of murine glioma cells engineered to express cb1 or cb2 receptors only when those receptors are coupled to akt activation18. Although a pro-tumourigenic effect has not been observed for the growth of tumour xenografts generated with glioma cells and treated with low doses of thc85, increased expression of amphiregulin promotes resistance to thc antitumour action through a mechanism that involves the egfr-dependent stimulation of erk and the subsequent inhibition of p8 and trb3 expression. Likewise, pharmacologic inhibition of egfr, erk83, or akt enhances the cell-death-promoting action of thc in glioma cultures (unpublished observations by the authors), which suggests that targeting egfr and the akt and erkpathways could enhance the antitumour effect of cannabinoids.
CANNABINOID-BASED COMBINATION THERAPIES
The use of combinational anticancer therapies has a number of theoretical advantages over single-agent strategies, because they allow for the simultaneous targeting of tumour growth, progression, and spread at various levels. In line with that idea, recent observations suggest that the combined administration of cannabinoids with other anticancer drugs acts synergistically to reduce tumour growth. For example, the administration of thc and temozolomide exerts strong antitumour action in glioma xenografts, an effect that is also evident in temozolomide-resistant tumours85. A similar effect was observed when thc and cbdwere combined with radiotherapy in animal models of glioma. Interestingly, no toxicity was observed in mice treated with combinations of thc and temozolomide85. Because most patients with glioblastoma undergo temozolomide treatment, the foregoing findings indicate that the combined administration of temozolomide and cannabinoids could be therapeutically exploited for the management of glioblastoma (Figure 2) and perhaps other tumour types such as melanoma44.
Likewise, another study recently showed that the combined administration of gemcitabine (the benchmark agent for the treatment of pancreatic cancer) and various cannabinoid agonists synergistically reduced the viability of pancreatic cancer cells88. Other reports indicated that anandamide and HU-210 might also enhance the anticancer activity of paclitaxel89 and 5-fluorouracil90 respectively.
An additional approach has been to combine thc with cbd, a phytocannabinoid that reduces (although to a lower extent than thc) the growth of several types of tumour xenografts through a still poorly-defined mechanism59,91,92. Combined administration of thc and cbd enhances the anticancer activity of thc and reduces the dose of thc needed to induce its tumour growth-inhibiting activity85,93. Moreover, the combination of thc and cbd together with temozolomide produces a striking reduction in the growth of glioma xenografts even when low doses of thc are used85. Likewise, the combination of thc, cbd, and radiotherapy also produced clear anticancer activity in an orthotopic model of glioma94. Notably, cbd was also shown to alleviate some of the undesired effects of thc administration such as convulsions, discoordination, and psychotic events, thus improving the tolerability of cannabis-based medicines15. As mentioned earlier, C. sativa produces approximately 108 different cannabinoids, and apart from cbd, some of the other cannabinoids present in marijuana might attenuate the psychoactive side effects of thc or even produce other therapeutic benefits15. Thus, we think that clinical studies aimed at analyzing the efficacy of cannabinoids as antitumour agents should be based on the use both of pure substances, such as thc and cbd, and of cannabis extracts containing controlled amounts of thc, cbd, and other cannabinoids.
CLINICAL ANTITUMOUR EFFECTS OF CANNABINOIDS
The clinical approval of cannabinoids is largely restricted to palliative uses in various diseases, but since the emergence of promising preclinical data, the antitumour effects of cannabinoids are beginning to be clinically assessed.
In a pilot phase i clinical study, 9 patients with actively- growing recurrent glioblastoma for whom standard therapy had previously failed underwent intracranial thc administration11. Under those conditions, cannabinoid delivery was safe and could be achieved without significant unwanted effects. In addition, although no statistically significant conclusions can be extracted from a cohort of 9 patients, the results obtained in the study suggest that some patients responded—at least partially—to thc treatment in terms of a decreased tumour growth rate as evaluated by magnetic resonance imaging11. Importantly, analyses of samples obtained from 2 study patients before and after thc administration indicated that the molecular mechanism of cannabinoid antitumour action—namely, p8 and trib3 upregulation35,37, mtorc1 inhibition37, stimulation of autophagy and apoptosis11,35,37, inhibition of cell proliferation11, decreased vegf signalling32, and matrix metalloproteinase 2 downregulation64 (delineated here earlier)—also operates in vivo.
Those findings were encouraging and reinforced interest in the potential use of cannabinoids in cancer therapies. However, they also highlighted the need for further research aimed at optimizing the use of cannabinoids in terms of patient selection, combination with other anti-cancer agents, and use of other routes of administration.
Administration of endocannabinoids or inhibitors of endocannabinoid-degrading enzymes has been shown to reduce the growth of various tumour xenograft types95,96 and could therefore be a reasonable strategy for targeting cannabinoid receptors for anticancer purposes. However, as discussed here earlier, the role of the endocannabinoid system, including the endocannabinoid-degrading enzymes, in the control of tumour generation and progression is not well understood. Because enhancing endocannabinoid tone only has mild antitumour effects in mice and because no inhibitor of endocannabinoid degradation has yet been approved for use in humans, clinical studies aimed at analyzing the efficacy of cannabinoids as antitumour agents should be based on the use of plant-derived or synthetic agonists of cannabinoid receptors rather than on endocannabinoids or inhibitors of endocannabinoid degradation.
The long-known therapeutic properties of C. sativa—including amelioration of symptoms associated with cancer and its chemotherapy—have led to the authorization of the medical use of the plant and its extracts in several countries. As already mentioned, C. sativa produces approximately 108 different cannabinoids, including thc and cbd. Some of the other cannabinoids present in marijuana might contribute to the attenuation of thc’s psychoactive side effects or even to the production of other therapeutic benefits15. However, pure drugs are more prone to standardization than complex molecular cocktails. Thus, it would be ideal for studies aiming to investigate the anticancer actions of cannabinoids in patients to be performed comparatively with both pure substances and cannabis extracts containing controlled amounts of thc, cbd, and other cannabinoids.
The most widely used route of administration for recreational and self-medicating marijuana is smoking. Although thc and other phytocannabinoids are rapidly absorbed by inhalation, smoking is an unattractive clinical option. Preclinical work in animal models has typically used peri-tumoural administration of cannabinoids. Likewise, in the only clinical trial in which a cannabinoid was assayed as an antitumour agent, thc was administered locally (intracranial delivery to patients with glioblastoma multiforme)11. Nevertheless, this route of administration has many obvious limitations. Currently available cannabis-based medicines are administered as capsules or using an oromucosal spray15. Preclinical animal models have yielded data indicating that systemic (oral or intraperitoneal) administration of cannabinoids effectively decreases tumour growth (GV, CS, and MG. Unpublished observations), and so it seems reasonable that future clinical studies with the goal of determining the efficacy of cannabinoids as antitumour agents use oral or oromucosal routes of administration.
Two currently ongoing clinical trials could shed some light on these issues. One of the studies is a phase i/iitrial aimed at evaluating the combined effect of Sativex (an oromucosal cannabis extract whose main active components are thc and cbd in a c. 1:1 ratio) and temozolomide in patients with recurrent glioblastoma multiforme (https://clinicaltrials.gov/ct2/show/NCT01812603 and https://clinicaltrials.gov/ct2/show/NCT01812616). The other is a phase ii trial aimed at evaluating the effect of cbd as single treatment in patients with solid tumours (https://clinicaltrials.gov/ct2/show/NCT02255292). Hopefully, in the near future, new clinical trials will start, helping to determine whether cannabinoids can be used, for other than their palliative effects, in the treatment of cancer patients
CONCLUSIONS AND FUTURE DIRECTIONS
It is widely believed that strategies aimed at reducing mortality from cancer should consist of targeted therapies capable of providing the most efficacious and selective treatment for each individual tumour and patient. Thus, the major focus of anticancer drug development has progressively moved from nonspecific chemotherapies to molecularly-targeted inhibitors. However, despite the huge amount of preclinical literature on how these rationally designed compounds work, their use in clinical practice is still limited.
How do cannabinoid-based medicines fit into this ongoing scenario? Consider glioma, the type of cancer in which the most detailed cannabinoid research has been conducted to date. As discussed here, engagement of a molecular target (the cb receptors) by a family of selective drugs (thc and other cannabinoid agonists) inhibits tumour growth in animal models through a well-established mechanism of action that also seems to operate in human patients. Moreover, cannabinoids potentiate the antitumour efficacy of temozolomide and alk inhibitors in mice harbouring gliomas. Those findings provide preclinical proof-of-concept that “cannabinoid sensitizers” could improve the clinical efficacy of classical cytotoxic drugs in glioblastoma (Figure 2) and perhaps other highly malignant tumours such as pancreatic cancer, melanoma, and hepatocellular carcinoma. However, further research is required to define the precise molecular cross-talk between cannabinoids and chemotherapeutic drugs and to optimize the pharmacology of preclinical cannabinoid-based combination therapies.
With respect to patient stratification, the particular individuals that are potentially responsive to cannabinoid administration should be unequivocally determined. To that end, high-throughput approaches should be implemented to find cannabinoid therapy–associated biomarkers in tumour biopsies or, ideally, in easily acquired fluids containing circulating cancer cells or enhanced levels of resistance factors that might have been released by cancer cells. Such biomarkers would conceivably relate to cannabinoid pharmacodynamics—namely, expression and activity of cannabinoid receptors and their downstream cell-death-inducing effectors. The approach would be analogous to the biochemical evaluation of estrogen and ErbB2 receptors, which respectively predict benefit from endocrine therapies and trastuzumab in breast cancer. Predictive markers to define the sensitivity of a particular tumour to cannabinoid-based therapies could also include the status of growth factors, such as mdk in gliomas, and their receptors and signalling partners.
To summarize, cannabinoids induce tumour cell death and inhibit tumour angiogenesis and invasion in animal models of cancer, and there are indications that they act similarly in patients with glioblastoma. Given that cannabinoids show an acceptable safety profile, clinical trials testing them as single drugs or, ideally, in combination therapies in glioblastoma and other types of cancer are both warranted and urgently needed.
Acknowledgments
Research by the authors and colleagues in their groups is supported by grants from the Spanish Ministry of Economy and Competitiveness and the Fondo Europeo de Desarrollo Regional (PI12/02248, PI15/0339, FR2009-0052, and IT2009-0053 to GV, and PI11/00295 to CS), Comunidad de Madrid (S2011/BMD-2308 to MG), GW Pharmaceuticals (to GV and CS), Fundación Mutua Madrileña (AP101042012 to GV), and Fundació La Marató de TV3 (20134031 to GV).
CONFLICT OF INTEREST DISCLOSURES
We have read and understood Current Oncology’s policy on disclosing conflicts of interest, and we declare the following interests: GW Pharmaceuticals and Cellmid fund part of the research conducted by our laboratory. Likewise, a portion of the data obtained by the authors concerning the antitumoural action of cannabinoids is included in three patent applications presented by GW Pharmaceuticals.
REFERENCES
1. Gaoni Y, Mechoulam R. Isolation, structure and partial synthesis of an active constituent of hashish. J Am Chem Soc. 1964;86:1646–7. doi: 10.1021/ja01062a046. [Cross Ref]
2. Pertwee RG. The diverse cb1 and cb2 receptor pharmacology of three plant cannabinoids: delta9-tetrahydrocannabinol, cannabidiol and delta9-tetrahydrocannabivarin. Br J Pharmacol. 2008;153:199–215. doi: 10.1038/sj.bjp.0707442. [PMC free article] [PubMed] [Cross Ref]
3. Devane WA, Hanus L, Breuer A, et al. Isolation and structure of a brain constituent that binds to the cannabinoid receptor. Science. 1992;258:1946–9. doi: 10.1126/science.1470919. [PubMed] [Cross Ref]
4. Mechoulam R, Ben-Shabat S, Hanus L, et al. Identification of an endogenous 2-monoglyceride, present in canine gut, that binds to cannabinoid receptors. Biochem Pharmacol. 1995;50:83–90. doi: 10.1016/0006-2952(95)00109-D. [PubMed] [Cross Ref]
5. Sugiura T, Kondo S, Sukagawa A, et al. 2-Arachidonoylglycerol: a possible endogenous cannabinoid receptor ligand in brain. Biochem Biophys Res Commun. 1995;215:89–97. doi: 10.1006/bbrc.1995.2437.[PubMed] [Cross Ref]
6. Pertwee RG, Howlett AC, Abood ME, et al. International Union of Basic and Clinical Pharmacology. LXXIX. Cannabinoid receptors and their ligands: beyond cb1 and cb2. Pharmacol Rev. 2010;62:588–631. doi: 10.1124/pr.110.003004. [PMC free article] [PubMed] [Cross Ref]
7. Matsuda LA, Lolait SJ, Brownstein MJ, Young AC, Bonner TI. Structure of a cannabinoid receptor and functional expression of the cloned cdna. Nature. 1990;346:561–4. doi: 10.1038/346561a0. [PubMed][Cross Ref]
8. Munro S, Thomas KL, Abu-Shaar M. Molecular characterization of a peripheral receptor for cannabinoids. Nature. 1993;365:61–5. doi: 10.1038/365061a0. [PubMed] [Cross Ref]
9. Fernandez-Ruiz J, Romero J, Velasco G, Tolon R, Ramos J, Guzman M. Cannabinoid cb2 receptor: a new target for controlling neural cell survival? Trends Pharmacol Sci. 2007;28:39–45. doi: 10.1016/j.tips.2006.11.001. [PubMed] [Cross Ref]
10. Atwood BK, Mackie K. cb2: a cannabinoid receptor with an identity crisis. Br J Pharmacol. 2010;160:467–79. doi: 10.1111/j.1476-5381.2010.00729.x. [PMC free article] [PubMed] [Cross Ref]
11. Guzman M, Duarte MJ, Blazquez C, et al. A pilot clinical study of delta9-tetrahydrocannabinol in patients with recurrent glioblastoma multiforme. Br J Cancer. 2006;95:197–203. doi: 10.1038/sj.bjc.6603236. [PMC free article] [PubMed] [Cross Ref]
12. Sarfaraz S, Adhami VM, Syed DN, Afaq F, Mukhtar H. Cannabinoids for cancer treatment: progress and promise. Cancer Res. 2008;68:339–42. doi: 10.1158/0008-5472.CAN-07-2785. [PubMed] [Cross Ref]
13. Katona I, Freund TF. Endocannabinoid signaling as a synaptic circuit breaker in neurological disease. Nat Med. 2008;14:923–30. doi: 10.1038/nm.f.1869. [PubMed] [Cross Ref]
14. Pacher P, Batkai S, Kunos G. The endocannabinoid system as an emerging target of pharmacotherapy. Pharmacol Rev. 2006;58:389–462. doi: 10.1124/pr.58.3.2. [PMC free article] [PubMed] [Cross Ref]
15. Pertwee RG. Emerging strategies for exploiting cannabinoid receptor agonists as medicines. Br J Pharmacol. 2009;156:397–411. doi: 10.1111/j.1476-5381.2008.00048.x. [PMC free article] [PubMed][Cross Ref]
16. Guzman M. Cannabinoids: potential anticancer agents. Nat Rev Cancer. 2003;3:745–55. doi: 10.1038/nrc1188. [PubMed] [Cross Ref]
17. Velasco G, Sanchez C, Guzman M. Towards the use of cannabinoids as antitumour agents. Nat Rev Cancer. 2012;12:436–44. doi: 10.1038/nrc3247. [PubMed] [Cross Ref]
18. Cudaback E, Marrs W, Moeller T, Stella N. The expression level of cb1 and cb2 receptors determines their efficacy at inducing apoptosis in astrocytomas. PloS One. 2010;5:e8702. doi: 10.1371/journal.pone.0008702. [PMC free article] [PubMed] [Cross Ref]
19. Hart S, Fischer OM, Ullrich A. Cannabinoids induce cancer cell proliferation via tumor necrosis factor alpha-converting enzyme (tace/adam17)–mediated transactivation of the epidermal growth factor receptor. Cancer Res. 2004;64:1943–50. doi: 10.1158/0008-5472.CAN-03-3720. [PubMed] [Cross Ref]
20. McKallip RJ, Nagarkatti M, Nagarkatti PS. Delta-9-tetrahydrocannabinol enhances breast cancer growth and metastasis by suppression of the antitumor immune response. J Immunol. 2005;174:3281–9. doi: 10.4049/jimmunol.174.6.3281. [PubMed] [Cross Ref]
21. Zhu LX, Sharma S, Stolina M, et al. Delta-9-tetrahydrocannabinol inhibits antitumour immunity by a cb2 receptor-mediated, cytokine-dependent pathway. J Immunol. 2000;165:373–80. doi: 10.4049/jimmunol.165.1.373. [PubMed] [Cross Ref]
22. Malfitano AM, Ciaglia E, Gangemi G, Gazzerro P, Laezza C, Bifulco M. Update on the endocannabinoid system as an anticancer target. Expert Opin Ther Targets. 2011;15:297–308. doi: 10.1517/14728222.2011.553606. [PubMed] [Cross Ref]
23. Sanchez C, de Ceballos ML, Gomez del Pulgar T, et al. Inhibition of glioma growth in vivo by selective activation of the cb2 cannabinoid receptor. Cancer Res. 2001;61:5784–9. [PubMed]
24. Caffarel MM, Sarrio D, Palacios J, Guzman M, Sanchez C. Delta9-tetrahydrocannabinol inhibits cell cycle progression in human breast cancer cells through Cdc2 regulation. Cancer Res. 2006;66:6615–21. doi: 10.1158/0008-5472.CAN-05-4566. [PubMed] [Cross Ref]
25. Nomura DK, Long JZ, Niessen S, Hoover HS, Ng SW, Cravatt BF. Monoacylglycerol lipase regulates a fatty acid network that promotes cancer pathogenesis. Cell. 2010;140:49–61. doi: 10.1016/j.cell.2009.11.027. [PMC free article] [PubMed] [Cross Ref]
26. Thors L, Bergh A, Persson E, et al. Fatty acid amide hydrolase in prostate cancer: association with disease severity and outcome, cb1 receptor expression and regulation by il-4. PLoS One. 2010;5:e12275. doi: 10.1371/journal.pone.0012275. [PMC free article] [PubMed] [Cross Ref]
27. Zheng D, Bode AM, Zhao Q, et al. The cannabinoid receptors are required for ultraviolet-induced inflammation and skin cancer development. Cancer Res. 2008;68:3992–8. doi: 10.1158/0008-5472.CAN-07-6594. [PMC free article] [PubMed] [Cross Ref]
28. Joosten M, Valk PJ, Jorda MA, et al. Leukemic predisposition of pSca-1/Cb2 transgenic mice. Exp Hematol. 2002;30:142–9. doi: 10.1016/S0301-472X(01)00779-2. [PubMed] [Cross Ref]
29. Perez-Gomez E, Andradas C, Blasco-Benito S, et al. Role of cannabinoid receptor cb2 in her2 pro-oncogenic signaling in breast cancer. J Natl Cancer Inst. 2015;107:djv077. doi: 10.1093/jnci/djv077.[PubMed] [Cross Ref]
30. Wang D, Wang H, Ning W, Backlund MG, Dey SK, DuBois RN. Loss of cannabinoid receptor 1 accelerates intestinal tumor growth. Cancer Res. 2008;68:6468–76. doi: 10.1158/0008-5472.CAN-08-0896. [PMC free article] [PubMed] [Cross Ref]
31. Izzo AA, Aviello G, Petrosino S, et al. Increased endocannabinoid levels reduce the development of precancerous lesions in the mouse colon. J Mol Med (Berl) 2008;86:89–98. doi: 10.1007/s00109-007-0248-4. [PMC free article] [PubMed] [Cross Ref]
32. Blazquez C, Gonzalez-Feria L, Alvarez L, Haro A, Casanova ML, Guzman M. Cannabinoids inhibit the vascular endothelial growth factor pathway in gliomas. Cancer Res. 2004;64:5617–23. doi: 10.1158/0008-5472.CAN-03-3927. [PubMed] [Cross Ref]
33. Galve-Roperh I, Sanchez C, Cortes ML, Gómez del Pulgar T, Izquierdo M, Guzman M. Antitumoral action of cannabinoids: involvement of sustained ceramide accumulation and extracellular signal-regulated kinase activation. Nat Med. 2000;6:313–19. doi: 10.1038/73171. [PubMed] [Cross Ref]
34. Gomez del Pulgar T, Velasco G, Sanchez C, Haro A, Guzman M. De novo–synthesized ceramide is involved in cannabinoid-induced apoptosis. Biochem J. 2002;363:183–8. doi: 10.1042/0264-6021:3630183. [PMC free article] [PubMed] [Cross Ref]
35. Carracedo A, Lorente M, Egia A, et al. The stress-regulated protein p8 mediates cannabinoid-induced apoptosis of tumor cells. Cancer Cell. 2006;9:301–12. doi: 10.1016/j.ccr.2006.03.005. [PubMed][Cross Ref]
36. Encinar JA, Mallo GV, Mizyrycki C, et al. Human p8 is a hmg-i/y–like protein with dna binding activity enhanced by phosphorylation. J Biol Chem. 2001;276:2742–51. doi: 10.1074/jbc.M008594200.[PubMed] [Cross Ref]
37. Salazar M, Carracedo A, Salanueva IJ, et al. Cannabinoid action induces autophagy-mediated cell death through stimulation of er stress in human glioma cells. J Clin Invest. 2009;119:1359–72. doi: 10.1172/JCI37948. [PMC free article] [PubMed] [Cross Ref]
38. Du K, Herzig S, Kulkarni RN, Montminy M. trb3: a tribbles homolog that inhibits Akt/pkb activation by insulin in liver. Science. 2003;300:1574–7. doi: 10.1126/science.1079817. [PubMed] [Cross Ref]
39. Verfaillie T, Salazar M, Velasco G, Agostinis P. Linking er stress to autophagy: potential implications for cancer therapy. Int J Cell Biol. 2010;2010:930509. doi: 10.1155/2010/930509. [PMC free article][PubMed] [Cross Ref]
40. Mizushima N, Levine B, Cuervo AM, Klionsky DJ. Autophagy fights disease through cellular self-digestion. Nature. 2008;451:1069–75. doi: 10.1038/nature06639. [PMC free article] [PubMed] [Cross Ref]
41. Eisenberg-Lerner A, Bialik S, Simon HU, Kimchi A. Life and death partners: apoptosis, autophagy and the cross-talk between them. Cell Death Differ. 2009;16:966–75. doi: 10.1038/cdd.2009.33. [PubMed][Cross Ref]
42. Galluzzi L, Pietrocola F, Bravo-San Pedro JM, et al. Autophagy in malignant transformation and cancer progression. EMBO J. 2015;34:856–80. doi: 10.15252/embj.201490784. [PMC free article] [PubMed][Cross Ref]
43. Vara D, Salazar M, Olea-Herrero N, Guzman M, Velasco G, Diaz-Laviada I. Antitumoral action of cannabinoids on hepatocellular carcinoma: role of ampk-dependent activation of autophagy. Cell Death Differ. 2011;18:1099–111. doi: 10.1038/cdd.2011.32. [PMC free article] [PubMed] [Cross Ref]
44. Armstrong JL, Hill DS, McKee CS, et al. Exploiting cannabinoid-induced cytotoxic autophagy to drive melanoma cell death. J Invest Dermatol. 2015;135:1629–37. doi: 10.1038/jid.2015.45. [PubMed][Cross Ref]
45. Ellert-Miklaszewska A, Kaminska B, Konarska L. Cannabinoids down-regulate pi3k/Akt and Erk signalling pathways and activate proapoptotic function of Bad protein. Cell Signal. 2005;17:25–37. doi: 10.1016/j.cellsig.2004.05.011. [PubMed] [Cross Ref]
46. Blazquez C, Carracedo A, Barrado L, et al. Cannabinoid receptors as novel targets for the treatment of melanoma. FASEB J. 2006;20:2633–5. doi: 10.1096/fj.06-6638fje. [PubMed] [Cross Ref]
47. Caffarel MM, Moreno-Bueno G, Cerutti C, et al. JunD is involved in the antiproliferative effect of delta9-tetrahydrocannabinol on human breast cancer cells. Oncogene. 2008;27:5033–44. doi: 10.1038/onc.2008.145. [PubMed] [Cross Ref]
48. Carracedo A, Gironella M, Lorente M, et al. Cannabinoids induce apoptosis of pancreatic tumor cells via endoplasmic reticulum stress-related genes. Cancer Res. 2006;66:6748–55. doi: 10.1158/0008-5472.CAN-06-0169. [PubMed] [Cross Ref]
49. Salazar M, Lorente M, Garcia-Taboada E, et al. The pseudokinase tribbles homologue-3 plays a crucial role in cannabinoid anticancer action. Biochim Biophys Acta. 2013;1831:1573–8. doi: 10.1016/j.bbalip.2013.03.014. [PubMed] [Cross Ref]
50. Caffarel MM, Andradas C, Perez-Gomez E, Guzman M, Sanchez C. Cannabinoids: a new hope for breast cancer therapy? Cancer Treat Rev. 2012;38:911–18. doi: 10.1016/j.ctrv.2012.06.005. [PubMed][Cross Ref]
51. Sarfaraz S, Afaq F, Adhami VM, Malik A, Mukhtar H. Cannabinoid receptor agonist-induced apoptosis of human prostate cancer cells LNCaP proceeds through sustained activation of erk1/2 leading to G1 cell cycle arrest. J Biol Chem. 2006;281:39480–91. doi: 10.1074/jbc.M603495200. [PubMed] [Cross Ref]
52. Guindon J, Hohmann AG. The endocannabinoid system and cancer: therapeutic implication. Br J Pharmacol. 2011;163:1447–63. doi: 10.1111/j.1476-5381.2011.01327.x. [PMC free article] [PubMed][Cross Ref]
53. Galve-Roperh I, Aguado T, Palazuelos J, Guzman M. Mechanisms of control of neuron survival by the endocannabinoid system. Curr Pharm Des. 2008;14:2279–88. doi: 10.2174/138161208785740117.[PubMed] [Cross Ref]
54. Casanova ML, Blazquez C, Martinez-Palacio J, et al. Inhibition of skin tumor growth and angiogenesis in vivo by activation of cannabinoid receptors. J Clin Invest. 2003;111:43–50. doi: 10.1172/JCI200316116.[PMC free article] [PubMed] [Cross Ref]
55. Chan PC, Sills RC, Braun AG, Haseman JK, Bucher JR. Toxicity and carcinogenicity of delta 9-tetrahydrocannabinol in Fischer rats and B6C3F1 mice. Fundam Appl Toxicol. 1996;30:109–17. doi: 10.1006/faat.1996.0048. [PubMed] [Cross Ref]
56. Lorente M, Torres S, Salazar M, et al. Stimulation of the midkine/alk axis renders glioma cells resistant to cannabinoid antitumoral action. Cell Death Differ. 2011;18:959–73. doi: 10.1038/cdd.2010.170.[PMC free article] [PubMed] [Cross Ref]
57. Ligresti A, Moriello AS, Starowicz K, et al. Antitumor activity of plant cannabinoids with emphasis on the effect of cannabidiol on human breast carcinoma. J Pharmacol Exp Ther. 2006;318:1375–87. doi: 10.1124/jpet.106.105247. [PubMed] [Cross Ref]
58. Massi P, Valenti M, Vaccani A, et al. 5-Lipoxygenase and anandamide hydrolase (faah) mediate the antitumor activity of cannabidiol, a non-psychoactive cannabinoid. J Neurochem. 2008;104:1091–100. doi: 10.1111/j.1471-4159.2007.05073.x. [PubMed] [Cross Ref]
59. Shrivastava A, Kuzontkoski PM, Groopman JE, Prasad A. Cannabidiol induces programmed cell death in breast cancer cells by coordinating the cross-talk between apoptosis and autophagy. Mol Cancer Ther. 2011;10:1161–72. doi: 10.1158/1535-7163.MCT-10-1100. [PubMed] [Cross Ref]
60. Nabissi M, Morelli MB, Santoni M, Santoni G. Triggering of the trpv2 channel by cannabidiol sensitizes glioblastoma cells to cytotoxic chemotherapeutic agents. Carcinogenesis. 2012;34:48–57. doi: 10.1093/carcin/bgs328. [PubMed] [Cross Ref]
61. Blazquez C, Casanova ML, Planas A, et al. Inhibition of tumor angiogenesis by cannabinoids. FASEB J. 2003;17:529–31. [PubMed]
62. Portella G, Laezza C, Laccetti P, De Petrocellis L, Di Marzo V, Bifulco M. Inhibitory effects of cannabinoid cb1 receptor stimulation on tumor growth and metastatic spreading: actions on signals involved in angiogenesis and metastasis. FASEB J. 2003;17:1771–3. [PubMed]
63. Pisanti S, Borselli C, Oliviero O, Laezza C, Gazzerro P, Bifulco M. Antiangiogenic activity of the endocannabinoid anandamide: correlation to its tumor-suppressor efficacy. J Cell Physiol. 2007;211:495–503. doi: 10.1002/jcp.20954. [PubMed] [Cross Ref]
64. Blazquez C, Salazar M, Carracedo A, et al. Cannabinoids inhibit glioma cell invasion by down-regulating matrix metalloproteinase-2 expression. Cancer Res. 2008;68:1945–52. doi: 10.1158/0008-5472.CAN-07-5176. [PubMed] [Cross Ref]
65. Grimaldi C, Pisanti S, Laezza C, et al. Anandamide inhibits adhesion and migration of breast cancer cells. Exp Cell Res. 2006;312:363–73. doi: 10.1016/j.yexcr.2005.10.024. [PubMed] [Cross Ref]
66. Qamri Z, Preet A, Nasser MW, et al. Synthetic cannabinoid receptor agonists inhibit tumor growth and metastasis of breast cancer. Mol Cancer Ther. 2009;8:3117–29. doi: 10.1158/1535-7163.MCT-09-0448.[PMC free article] [PubMed] [Cross Ref]
67. Preet A, Ganju RK, Groopman JE. Delta9-tetrahydrocannabinol inhibits epithelial growth factor-induced lung cancer cell migration in vitro as well as its growth and metastasis in vivo. Oncogene. 2008;27:339–46. doi: 10.1038/sj.onc.1210641. [PubMed] [Cross Ref]
68. Ramer R, Hinz B. Inhibition of cancer cell invasion by cannabinoids via increased expression of tissue inhibitor of matrix metalloproteinases-1. J Natl Cancer Inst. 2008;100:59–69. doi: 10.1093/jnci/djm268.[PubMed] [Cross Ref]
69. McAllister SD, Murase R, Christian RT, et al. Pathways mediating the effects of cannabidiol on the reduction of breast cancer cell proliferation, invasion, and metastasis. Breast Cancer Res Treat. 2011;129:37–47. doi: 10.1007/s10549-010-1177-4. [PMC free article] [PubMed] [Cross Ref]
70. Soroceanu L, Murase R, Limbad C, et al. Id-1 is a key transcriptional regulator of glioblastoma aggressiveness and a novel therapeutic target. Cancer Res. 2012;73:1559–69. doi: 10.1158/0008-5472.CAN-12-1943. [PMC free article] [PubMed] [Cross Ref]
71. Newton CA, Chou PJ, Perkins I, Klein TW. cb1 and cb2 cannabinoid receptors mediate different aspects of delta-9-tetrahydrocannabinol (thc)–induced T helper cell shift following immune activation by Legionella pneumophila infection. J Neuroimmune Pharmacol. 2009;4:92–102. doi: 10.1007/s11481-008-9126-2. [PubMed] [Cross Ref]
72. Lu T, Newton C, Perkins I, Friedman H, Klein TW. Cannabinoid treatment suppresses the T-helper cell-polarizing function of mouse dendritic cells stimulated with Legionella pneumophila infection. J Pharmacol Exp Ther. 2006;319:269–76. doi: 10.1124/jpet.106.108381. [PubMed] [Cross Ref]
73. Steffens S, Veillard NR, Arnaud C, et al. Low dose oral cannabinoid therapy reduces progression of atherosclerosis in mice. Nature. 2005;434:782–6. doi: 10.1038/nature03389. [Erratum in: Nature 2005;435:528] [PubMed] [Cross Ref]
74. Hegde VL, Nagarkatti M, Nagarkatti PS. Cannabinoid receptor activation leads to massive mobilization of myeloid-derived suppressor cells with potent immunosuppressive properties. Eur J Immunol. 2010;40:3358–71. doi: 10.1002/eji.201040667. [PMC free article] [PubMed] [Cross Ref]
75. Burstein SH, Zurier RB. Cannabinoids, endocannabinoids, and related analogs in inflammation. AAPS J. 2009;11:109–19. doi: 10.1208/s12248-009-9084-5. [PMC free article] [PubMed] [Cross Ref]
76. Liu WM, Fowler DW, Dalgleish AG. Cannabis-derived substances in cancer therapy—an emerging anti-inflammatory role for the cannabinoids. Curr Clin Pharmacol. 2010;5:281–7. doi: 10.2174/157488410793352049. [PubMed] [Cross Ref]
77. Mirkin BL, Clark S, Zheng X, et al. Identification of midkine as a mediator for intercellular transfer of drug resistance. Oncogene. 2005;24:4965–74. doi: 10.1038/sj.onc.1208671. [PubMed] [Cross Ref]
78. Kadomatsu K. The midkine family in cancer, inflammation and neural development. Nagoya J Med Sci. 2005;67:71–82. [PubMed]
79. Palmer RH, Vernersson E, Grabbe C, Hallberg B. Anaplastic lymphoma kinase: signalling in development and disease. Biochem J. 2009;420:345–61. doi: 10.1042/BJ20090387. [PMC free article][PubMed] [Cross Ref]
80. Lonardi S, Tosoni A, Brandes AA. Adjuvant chemotherapy in the treatment of high grade gliomas. Cancer Treat Rev. 2005;31:79–89. doi: 10.1016/j.ctrv.2004.12.005. [PubMed] [Cross Ref]
81. Nieder C, Adam M, Molls M, Grosu AL. Therapeutic options for recurrent high-grade glioma in adult patients: recent advances. Crit Rev Oncol Hematol. 2006;60:181–93. doi: 10.1016/j.critrevonc.2006.06.007. [PubMed] [Cross Ref]
82. Purow B, Schiff D. Advances in the genetics of glioblastoma: are we reaching critical mass? Nat Rev Neurol. 2009;5:419–26. doi: 10.1038/nrneurol.2009.96. [PMC free article] [PubMed] [Cross Ref]
83. Lorente M, Carracedo A, Torres S, et al. Amphiregulin is a factor for resistance of glioma cells to cannabinoid-induced apoptosis. Glia. 2009;57:1374–85. doi: 10.1002/glia.20856. [PubMed] [Cross Ref]
84. Stupp R, Mason WP, van den Bent MJ, et al. Radiotherapy plus concomitant and adjuvant temozolomide for glioblastoma. N Engl J Med. 2005;352:987–96. doi: 10.1056/NEJMoa043330. [PubMed][Cross Ref]
85. Torres S, Lorente M, Rodriguez-Fornes F, et al. A combined preclinical therapy of cannabinoids and temozolomide against glioma. Mol Cancer Ther. 2011;10:90–103. doi: 10.1158/1535-7163.MCT-10-0688.[PubMed] [Cross Ref]
86. de Bono JS, Ashworth A. Translating cancer research into targeted therapeutics. Nature. 2010;467:543–9. doi: 10.1038/nature09339. [PubMed] [Cross Ref]
87. Grande E, Bolos MV, Arriola E. Targeting oncogenic alk: a promising strategy for cancer treatment. Mol Cancer Ther. 2011;10:569–79. doi: 10.1158/1535-7163.MCT-10-0615. [PubMed] [Cross Ref]
88. Donadelli M, Dando I, Zaniboni T, et al. Gemcitabine/cannabinoid combination triggers autophagy in pancreatic cancer cells through a ros-mediated mechanism. Cell Death Dis. 2011;2:e152. doi: 10.1038/cddis.2011.36. [PMC free article] [PubMed] [Cross Ref]
89. Miyato H, Kitayama J, Yamashita H, et al. Pharmacological synergism between cannabinoids and paclitaxel in gastric cancer cell lines. J Surg Res. 2009;155:40–7. doi: 10.1016/j.jss.2008.06.045. [PubMed][Cross Ref]
90. Gustafsson SB, Lindgren T, Jonsson M, Jacobsson SO. Cannabinoid receptor–independent cytotoxic effects of cannabinoids in human colorectal carcinoma cells: synergism with 5-fluorouracil. Cancer Chemother Pharmacol. 2009;63:691–701. doi: 10.1007/s00280-008-0788-5. [PubMed] [Cross Ref]
91. Massi P, Vaccani A, Bianchessi S, Costa B, Macchi P, Parolaro D. The non-psychoactive cannabidiol triggers caspase activation and oxidative stress in human glioma cells. Cell Mol Life Sci. 2006;63:2057–66. doi: 10.1007/s00018-006-6156-x. [PubMed] [Cross Ref]
92. McAllister SD, Christian RT, Horowitz MP, Garcia A, Desprez PY. Cannabidiol as a novel inhibitor of Id-1 gene expression in aggressive breast cancer cells. Mol Cancer Ther. 2007;6:2921–7. doi: 10.1158/1535-7163.MCT-07-0371. [PubMed] [Cross Ref]
93. Marcu JP, Christian RT, Lau D, et al. Cannabidiol enhances the inhibitory effects of delta9-tetrahydrocannabinol on human glioblastoma cell proliferation and survival. Mol Cancer Ther. 2010;9:180–9. doi: 10.1158/1535-7163.MCT-09-0407. [PMC free article] [PubMed] [Cross Ref]
94. Scott KA, Dalgleish AG, Liu WM. The combination of cannabidiol and Delta9-tetrahydrocannabinol enhances the anticancer effects of radiation in an orthotopic murine glioma model. Mol Cancer Ther. 2014;13:2955–67. doi: 10.1158/1535-7163.MCT-14-0402. [PubMed] [Cross Ref]
95. Bifulco M, Laezza C, Portella G, et al. Control by the endogenous cannabinoid system of ras oncogene-dependent tumor growth. FASEB J. 2001;15:2745–7. [PubMed]
96. Ligresti A, Bisogno T, Matias I, et al. Possible endocannabinoid control of colorectal cancer growth. Gastroenterology. 2003;125:677–87. doi: 10.1016/S0016-5085(03)00881-3. [PubMed] [Cross Ref]